Tuesday Sept. 4, 2007
An iron bar was passed around class at the beginning of class.
Students were asked to guess how much it weighed.

Air in Tucson dried out dramatically over the weekend. Dew
points, which had been in the 60s since the start of the summer monsoon
in early July dropped into the upper 30s. Tropical moisture from
the remains of Hurricane Henriette are expected to move into Arizona by
mid week and should increase our chances of rain. You can track
the progress of Hurricane Henriette and also Hurricane Felix (which
made landfall on the east coast of Central America this morning) at the
National Hurricane Center website.
The latest forecast for Tucson and Southern Arizona can be found at the
National Weather Service Tucson
Forecast Office website
I forgot to mention that the first of this semester's 1S1P assignments is now online. I will
discuss this in class before the Practice Quiz this coming
Thursday.
A copy of the Practice Quiz Study Guide was handed out in class.
Reviews are scheduled for Tue. and Wed. afternoon from 4 - 5 pm in FCS
225.

There's a lot of information in this figure. The atmosphere
that
surrounds the earth has mass. Gravity pulls downward on the
air giving it weight. Galileo used a simple
demonstration to prove that air has weight.
Pressure is defined as force divided by area; in this case the weight
of the atmosphere divided by area. Atmospheric pressure is
determined by and tells you something about the weight of the air
overhead.
Under normal conditions a 1 inch by 1 inch column of air stretching
from sea level to the top of the atmosphere will weigh 14.7
pounds. That is the same as the steel bar that was passed around
class (many people think it is heavier than 14.7 pounds). Normal
atmospheric
pressure at sea level
is 14.7 pounds per square inch (psi). Psi are the pressure units
you use when you fill up
your car or bike tires with air.
We will mostly use millibar (mb) units in this course.
Standard
atmospheric
pressure is about 1000 mb or 30 inches of mercury. The second
value refers to the reading from a mercury barometer. 1000
millibars is also equal to 1 bar or 1 atmosphere.
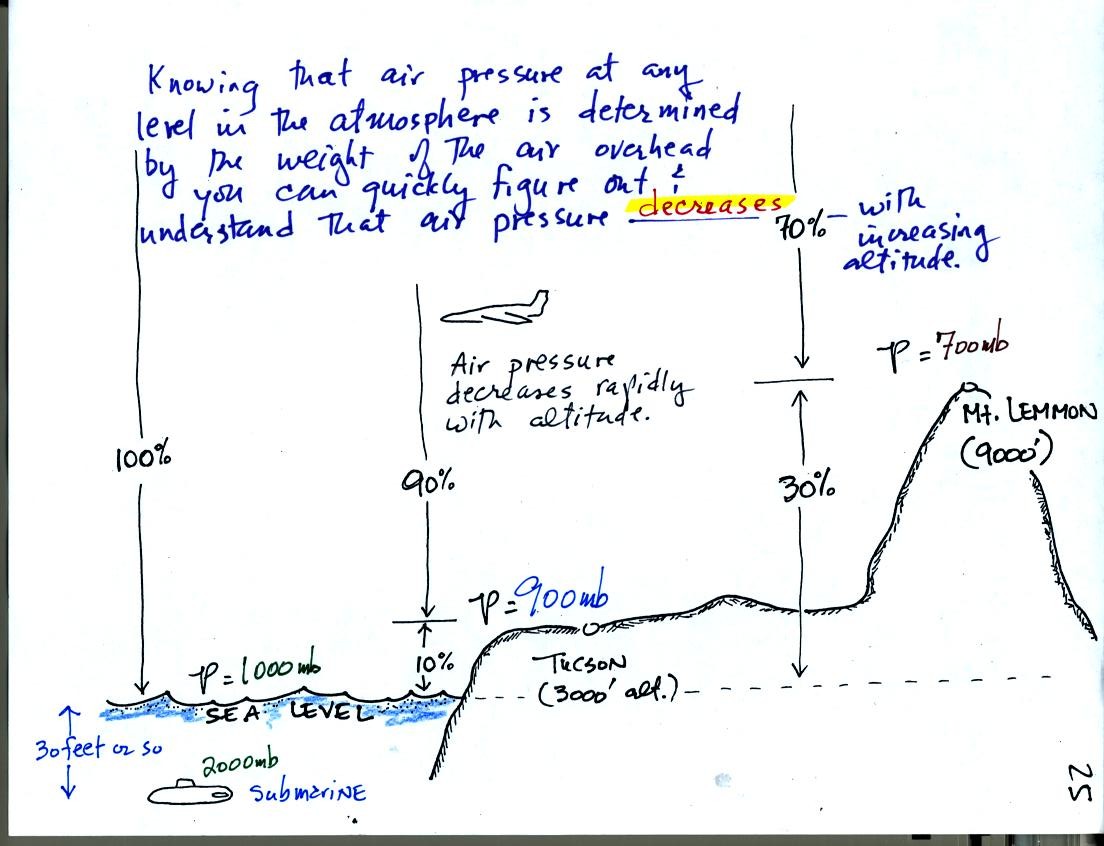
As you move upward through the atmosphere there is less and
less air
left overhead. The pressure at any level in the atmosphere is
determined by the weight of the air remaining overhead. Thus pressure
decreases with increasing altitude.
Pressure changes much more
quickly when you move in a vertical direction than it does when you
move horizontally. This will be important when we cover surface
weather maps. Meterologists attempt to map out small horizontal
changes or differences in pressure on weather maps. These small
changes are what cause the wind to blow and produce weather.
Pressure increases even more rapidly as you descend into the
ocean. The
pressure at some level in the ocean is determined by the atmospheric
pressure plus the pressure produced by the weight of the water above
you. Water is much denser than air, so the extra weight builds up
quickly. The submarine in the lower
left hand corner of the figure above would experience a pressure of
2000
mb at 30 feet in the ocean, twice what it would feel at the surface of
the ocean. Only 30 feet of water has produced the same pressure
as the entire atmosphere.
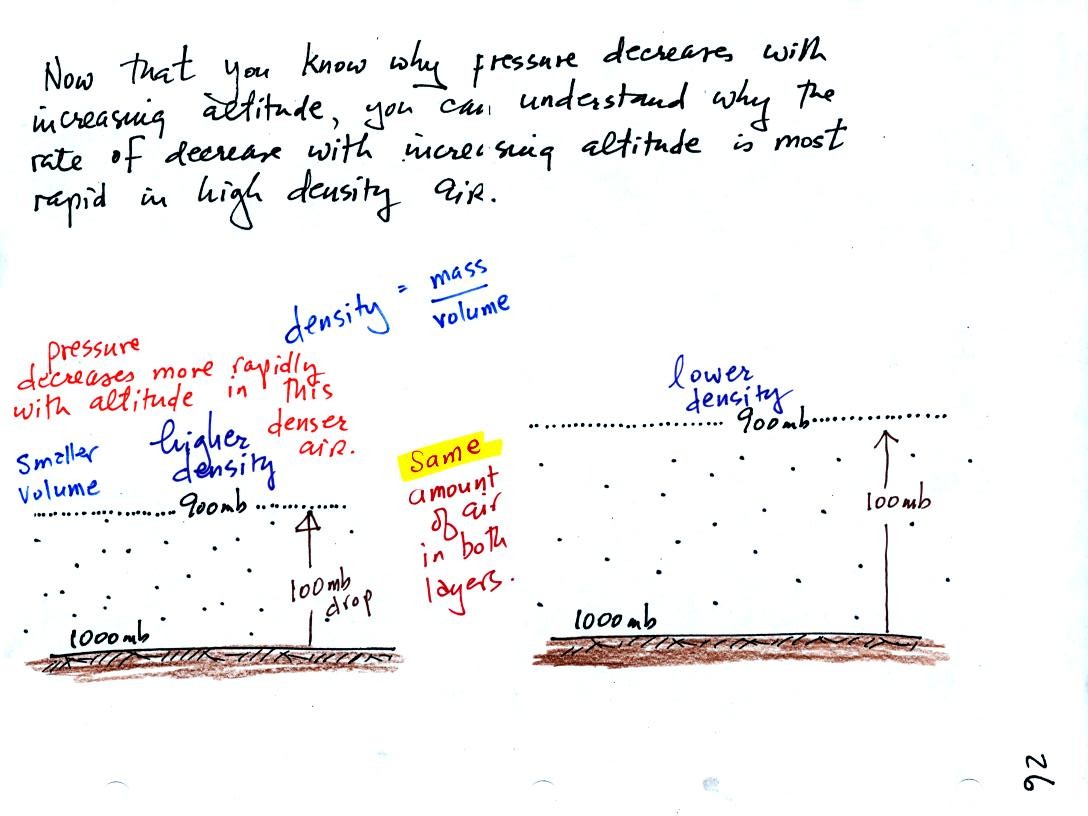
As you move upward from the ground pressure decreases by 100
mb in both
layers in the figure above. Both layers contain the same amount
of air (if you refer
back to the previous figure you will find the a 100 mb drop when you go
from sea level to Tucson - 10% of the
air in the atmosphere lies between sea level and 3000 ft altitude in
Tucson). That air is found in a smaller volume in
the figure at left (the layer is thinner). This means the air at
left is denser than the air at right. The drop in air pressure in
the layer at left occurs in a shorter vertical distance than in the air
layer at right. That is a more rapid rate of pressure decrease
with distance than in the layer at right.
The rate of pressure decrease
with altitude is higher in the dense air at left than in the lower
density air at right.
This is a fairly subtle but important concept. We will use this
concept later in the semester when we try to understand the
intensification of hurricanes.
The
following discussion of Newton's Law of Universal Gravitation wasn't
covered in class.
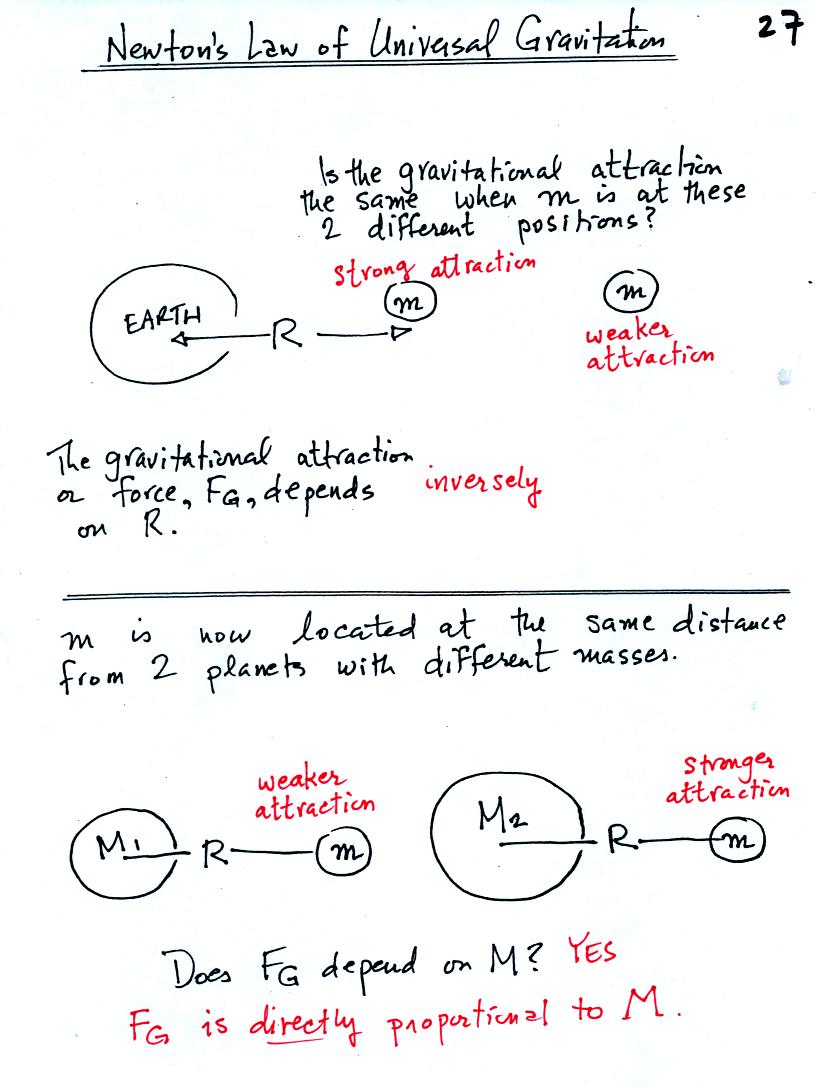
The gravitational attraction between two objects depends first of
all
on the distance separating the objects. The gravitational
force becomes weaker the further away the two objects are from each
other. In the bottom
picture above and the top figure below we see that the attractive force
also depends on the masses of the two objects.
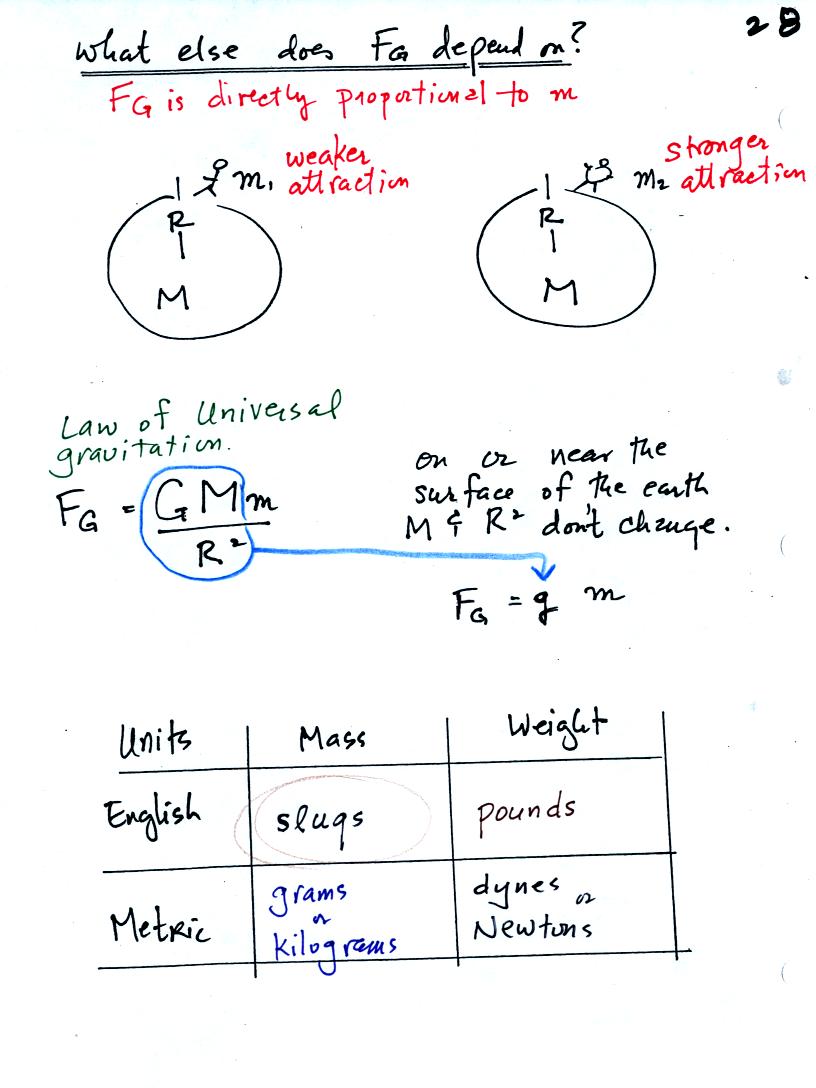
The complete formula is shown in the middle of the page
above. G
is a constant. On the surface of the earth G, M, and R don't
change. The gravitational acceleration, g, is just G times Mearth
divided by ( Rearth )2
. To determine the weight (on the earth's surface) of an object
with mass m you simply multiply m x g.
Down at the bottom of the page are the Metric and English units of mass
and weight.
Here's another page from the
photocopied Class Notes that we didn't cover in class. The
weight of a person on the earth and the moon is calculated in English
and metric units.
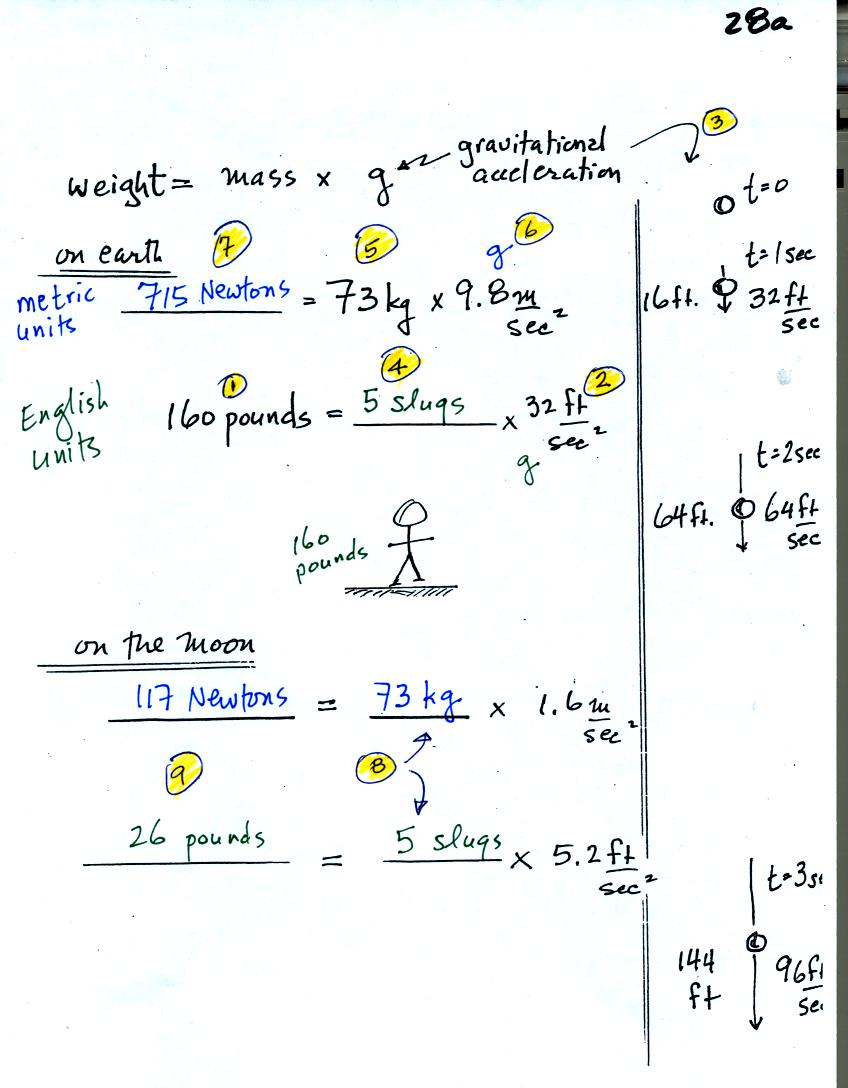
(1) The course instructor weighs about 160 pounds. In
(2) we see that the
gravitational acceleration is 32 ft/sec2 in English units. The
meaning of this value is shown in (3). Gravity will cause a
falling object to fall 32 ft/sec faster with every second it continues
to fall. Dividing the instructor's weight by the gravitation
acceleration in (4) we obtain the instructor's mass, 5 slugs, in
English units.
In metric units, the instructor has a mass of 73 kilograms (5).
The gravitation acceleration is 9.8 m/sec (6). Multiplying these
two values, in (7), we find that the instructor weigh 715 Newtons.
On the moon, the mass stays the same. Gravity is weaker, so the
value of g is smaller. The instructor would weigh quite a bit
less (117 Newtons or 26 pounds) on the moon compared to the earth.

Air pressure is a force that pushes downward, upward, and
sideways.
If you fill a balloon with air and then push downward on it, you can
feel the air in the balloon pushing back (pushing upward). You'd
see the air in the balloon pushing sideways as well.
The bottom person in the people pyramid below must push upward with
enough
force to support the other people. The air in a layer at the
bottom of the atmosphere must do the same thing. It pushes upward
with enough force to support the weight of all the air overhead.
The air
pressure in the four tires on your automobile push down on the road
(that's something you would feel if the car ran over your foot) and
push upward
with enough force to keep the 1000 or 2000 pound vehicle off the
road.
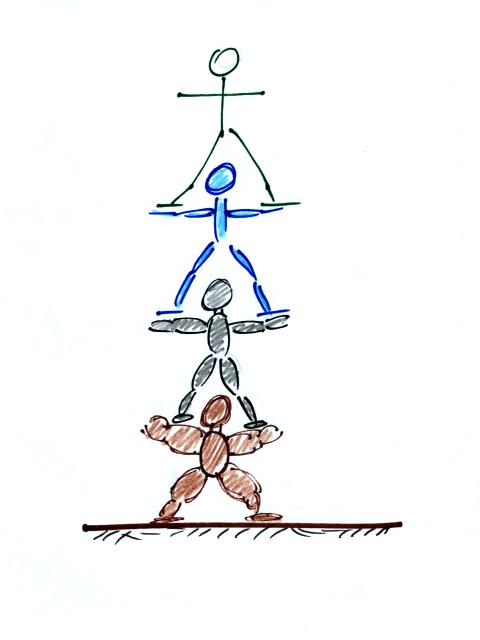
The person on the bottom of the "people pyramid"
must
support
the weight of all the people above. People in the middle don't
have to support as much weight.
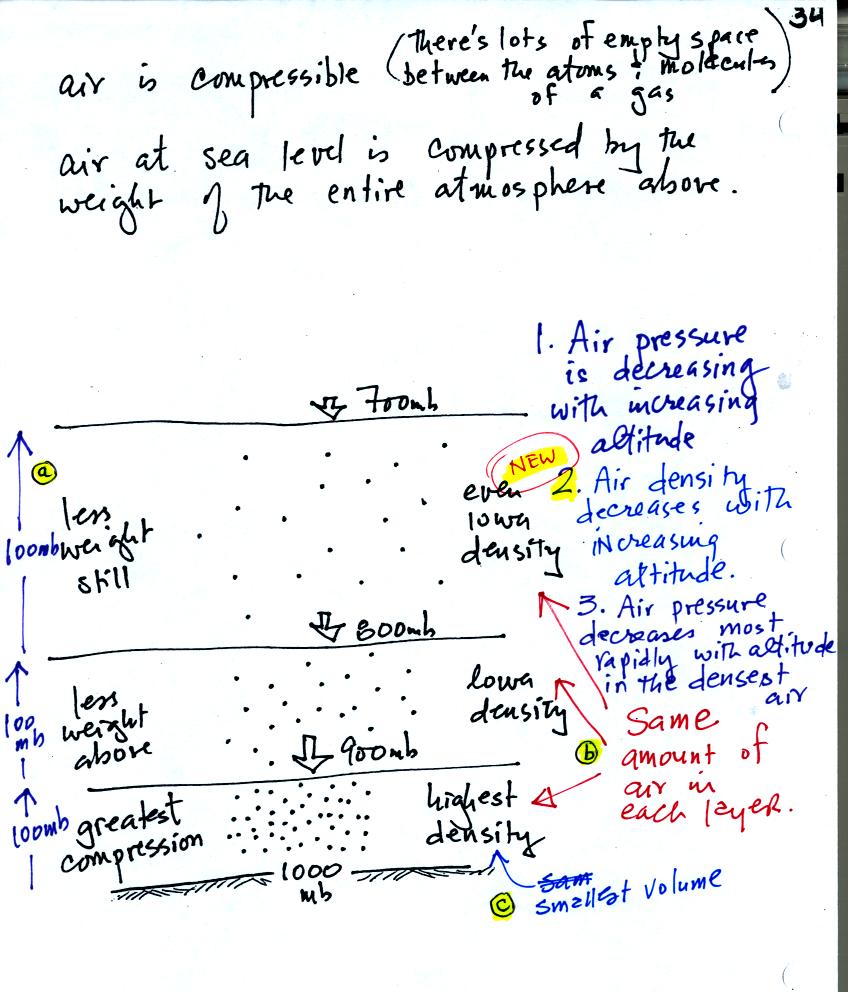
Three layers of air in the atmosphere are
shown above (point b: each
layer
contains the same amount of air, 10% of the air in the
atmosphere). You can tell because you see the same pressure drop
as you move upward through each layer (point a). This picture
reminds you that air
pressure decreases with
increasing altitude.
The layer at the ground and at the bottom of the
atmosphere is "squished" by the weight of the air above.
Squeezing all of this air into a thin layer or small volume (point c)
increases
the air's density. The highest air density is found at the bottom
of the atmosphere.
The next layer up is also squished but not as much as the bottom
layer. The density of the air in the second layer is lower than
in the bottom layer. The air in the 3rd layer has even lower
density. It is fairly easy to understand that air density
decreases with increasing altitude.
Finally if you look closely at the figure you can see that pressure decreases most rapidly
with increasing altitude in the dense air at the bottom of the
atmosphere.
We
finished class with a demonstration of the upward force caused by air
pressure.
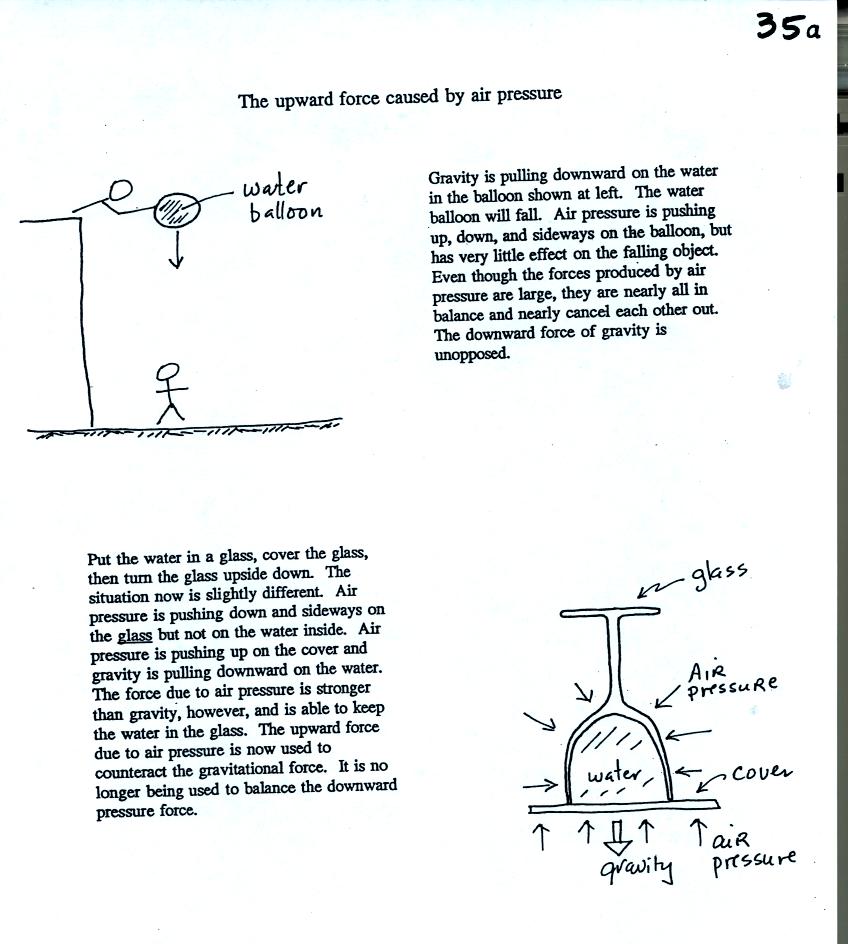
The demonstration is described on p. 35a in
the photocopied Class Notes.
The following more detailed look at
what is going on in the demonstration wasn't covered in class
. First
the case of a water balloon:

The figure at left shows air pressure (red
arrows)
pushing on all
the
sides of the balloon. Because pressure decreases with increasing
altitude, the pressure pushing downward on the top of the balloon is a
little weaker (strength=14) than the pressure pushing upward at the
bottom of the
balloon (strength=15). The two sideways forces cancel each other
out. The
total effect of the pressure is a weak upward force (shown on the right
figure, you might have heard this called a bouyant force).
Gravity exerts a downward force on the water
balloon. In the figure at right you can see that the gravity
force (strength=10) is stronger than the upward pressure difference
force (strength=1). The
balloon falls as a result.
In the demonstration a wine glass is filled with water. A small
plastic lid is used to cover the wine glass. You can then turn
the glass upside down without the water falling out.
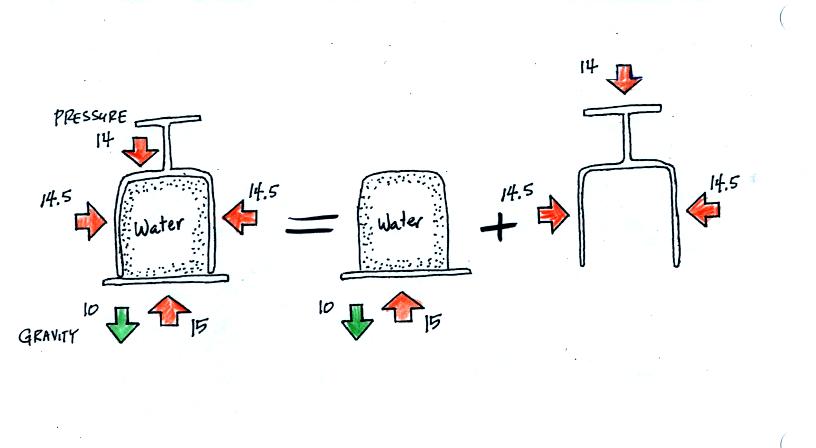
All the same forces are shown again in the left most
figure.
In
the right two figures we separate this into two parts. First
the water inside the glass isn't feeling the downward and sideways
pressure forces (because they're pushing on the glass). Gravity
still pulls downward on the water but the upward pressure force is able
to overcome the downward pull of gravity. The upward pointing
pressure force is used to overcome gravity not to cancel out the
downward pointing pressure force.
The demonstration was repeated using a 4 Liter flash (more than a
gallon of water, more than 8 pounds of water). The upward
pressure force was still able to keep the water in the flask (much of
the weight of the water is pushing against the sides of the flask which
the instructor was supporting with his arms).