Tuesday Oct. 6, 2009
click here to download today's notes in
a more printer friendly format
A couple of songs ( " "My Song," and "The Story" ) from Brandi Carlile
before class today. She'll be in Tucson at the Rialto Theatre on Fri., Oct.
16.
The 1S1P Assignment #1 Topic #2 reports were collected today. I
am hoping to return the Radon reports in class on Thursday.
The first part of the Quiz
#2 Study Guide
is
now available online. By the time today's notes are online, the
complete study guide (in preliminary form) should be available.
Optional Assignment #1 was returned in class today. If you don't
see a grade marked on your paper it means you earned full credit (0.5
extra credit points).
The Experiment
#2 reports are due next Tuesday (Oct. 13). Please
return your materials this week and pick up the supplementary
information sheet. You can bring them to class or drop them off
in my office (PAS 588).
In the next week or so we will be learning about several different
forms of energy, energy transport, and the atmospheric greenhouse
effect. Class started with a little bit of an overview before getting into the details.
When you
add energy to an object, the object will usually
warm
up (conversely when you take energy from an object the object will
cool). It is relatively easy to come up with an equation that
allows
you to figure out what the temperature change will be.
The temperature change will
first depend on
how much energy was added. This is a direct proportionality, so
delta E is in the numerator of the
equation (delta E and delta T are both positive when energy is added,
negative when energy is taken from something)/
When you add equal amounts of energy to large and small pans
of water, the small pan will heat up more
quickly. The temperature change, delta T, will depend on the
mass. A small mass will mean a large delta T, so mass should go
in the denominator of the equation.
Different materials
react differently when energy is added to them. A material with a
large specific heat will warm more slowly than a material with a small
specific heat. Specific heat has the same kind of effect on delta
T as
mass. Specific heat is sometimes called "thermal mass" or
"thermal capacity."
Here's an important example that will show the effect of specific
heat (middle of p. 45)
Equal
amounts of energy (1000 calories, note that calories are units of
energy) are added to
equal masses (200 grams) of water and soil. We use water and soil
in the
example because most of the earth's surface is either ocean or land.
Water has a higher specific heat than soil, it only warms up 5o
C.
The soil has a lower specific heat and warms up 25o C, 5
times more
than the water.
These different rates of warming of water and soil have
important effects on regional climate.
Oceans moderate the climate.
Cities near a large body
of water won't warm as much in the summer and won't cool as much during
the winter compared to a city that is surrounded by land.
The city above on the
coast has a 30o F annual range of temperature (range is the
difference between the summer and winter temperatures). The
city further
inland (assumed to be at the same latitude and altitude) has an annual
range of 60o F. Note that both cities have the same 60o
F annual
mean temperature.
Adding
energy to an object will usually cause it to warm. But there
is another possibility (bottom p. 45), the object could change
phase (change
from solid to liquid or
gas). Adding energy to ice might cause
the
ice to melt. Adding energy to water could cause it to evaporate.
The equation at the bottom of the figure above allows you to
calculate how much energy is required to melt ice or evaporate water or
sublimate dry ice. You multiply the mass by the latent heat, a
variable that depends on the particular material that is changing
phase.
If you add energy to or remove energy from an object, the
object
will usually change temperature. You can calculate the
temperature change if you know the object's mass and its specific
heat.
We will be using the equation in a slightly different way in a class
experiment/demonstration. We will measure the temperature change and
use that to
determine the amount of energy lost by an object.
The object of the experiment was to
measure the latent heat of
vaporization of liquid nitrogen. That just means measuring the
amount of energy needed to evaporate a gram of liquid nitrogen.
The students that are doing Experiment #2 are measuring the latent heat
of fusion of ice, the energy needed to melt one gram of ice.
You'll
find the following figure on p. 45a in the photocopied
Classnotes.
(a)
Some room temperature water poured into a styrofoam cup weighed
155
g. The cup itself weighed 3.8 g, so we had 151.2 g of water.
(b)
The water's temperature was 20.8 C (room temperature).
(c)
44.0 g of liquid nitrogen was poured into the cup of water.
It takes energy to turn liquid nitrogen into nitrogen gas.
The needed energy came from the water. This flow of energy is
shown in the middle figure above. We assumed that because the
experiment is performed in a styrofoam cup that there is no energy
flowing between the water in the cup and the surounding air.
(d)
After the liquid nitrogen had evaporated we remeasured the water's
temperature. It had dropped to 6.2 C. That is a
temperature drop of 20.8 - 6.2 = 14.6 C.
Because we knew how
much water we started with, its temperature drop, and water's specific
heat we can calculate how much
energy was taken from the water. That is the 2207.5 calorie
figure above. This was used to evaporate 44 grams of liquid
nitrogen. So we divided 2207.5 calories by 44 grams to get 50.2
calories needed per gram. That is our
measured value of the latent heat of vaporization of nitrogen. A
trustworthy student in the class informed us that
the known value is 48 cal/g, so our measurement
was pretty darn close.
When you
add energy to an object and the object warms, what exactly is
happening inside the object?
The figure above is on p. 46 in the
photocopied Class
Notes. Temperature provides a measure of the average kinetic of the
atoms or
molecules in a material. The atoms or molecules in a cold
material will be moving more slowly than the atoms or molecules in a
warmer object.
You need to be careful what temperature scale you use when
using
temperature as a measure of average kinetic energy. You must
use the Kelvin temperature scale because it does not go
below zero (0 K is known as absolute zero). The smallest kinetic
energy you can have is zero
kinetic energy. There is no such thing as negative kinetic energy.
You can think of heat as being the total kinetic energy of all
the
molecules or atoms in a material.
The next figure might make the distinction between temperature (average
kinetic energy) and heat (total kinetic energy) clearer.
A cup of water and a pool of water
both have the same
temperature. The average kinetic energy of the water molecules in
the pool and in the cup are the same. There are a lot more
molecules in the pool than in the cup. So if you add together all
the kinetic
energies of all the molecules in the pool you are going to get a much
bigger number than if you sum the kinetic energies of the molecules in
the cup. There is
a lot more stored energy in the pool than in the cup. It would be
a lot harder to cool (or warm) all the water in the pool than it would
be the cup.
In the same way the two groups of people shown have the same
average
amount
of money per person (that's analogous to temperature). The $100
held by the larger group at the
left is
greater than the $20 total possessed by the smaller group of people on
the right (total amount of money is analogous to heat).
Speaking
of temperature scales.
You should remember the
temperatures of the boiling point
and freezing
point of water on the Fahrenheit, Celsius, and perhaps the Kelvin
scales. 300
K is a
good easy-to-remember value for the global annual average surface
temperature of the earth.
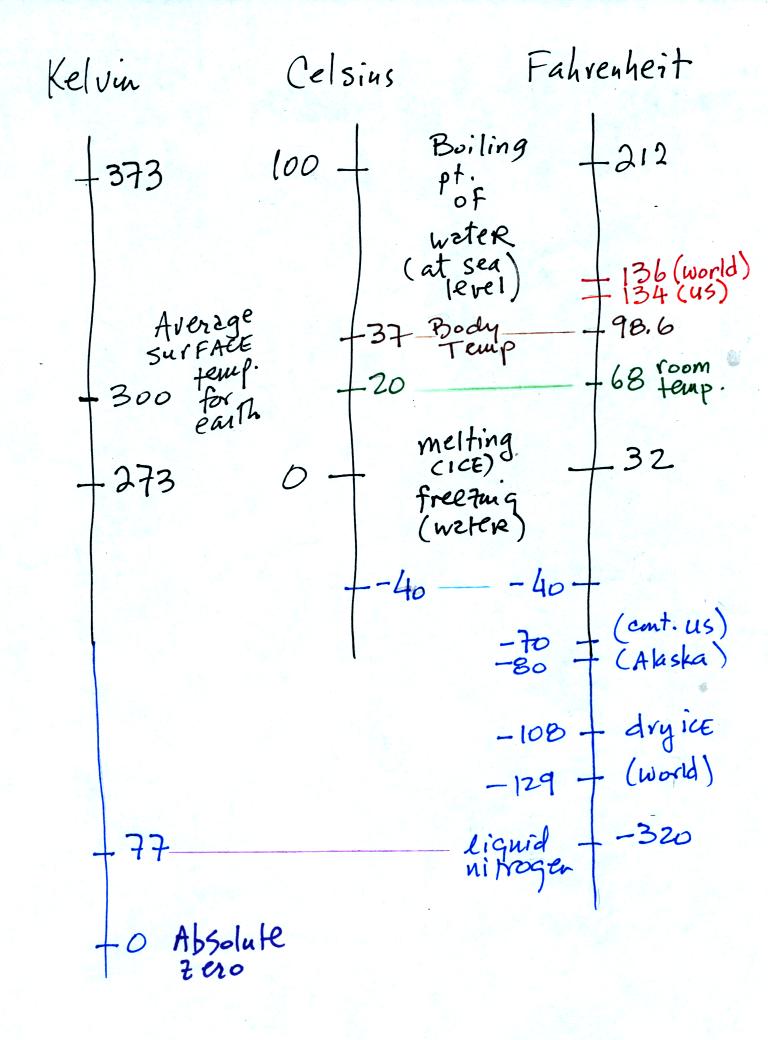
You certainly don't need to try to
remember all these
numbers. The world high temperature record was set in Libya, the
US
record in
Death Valley. The continental US cold temperature record of -70 F
was set in Montana and the -80 F value in Alaska. The world
record -129 F was measured at Vostok station in Antarctica. This
unusually cold reading was the result of three factors: high latitude,
high altitude, and location in the middle of land rather than being
near or
surrounded by ocean. Liquid
nitrogen is cold but it is still quite a bit warmer than absolute zero.
Conduction
is the first of four energy transport processes
that we
will cover (the least important transport process in the
atmosphere). The figure below illustrates this process. A
hot object is stuck in the middle of some air.
In the top picture some of the
atoms or molecules near the
hot object have collided with the object and picked up energy from the
object. This is reflected by the increased speed
of motion or increased kinetic energy of these molecules or
atoms (these guys are colored pink).
In the middle picture the
initial bunch of
energetic molecules have
collided with some of their neighbors and shared energy with
them (these are orange). The neighbor molecules have gained
energy though they don't
have as much energy as the molecules next to the hot object.
In
the third picture molecules further out have now (the yellow ones)
gained
some energy. The random motions and collisions
between molecules
is carrying energy from the hot object out into the colder material.
Conduction transports energy from hot to cold. The rate of
energy transport depends first on the material (air in the example
above). Thermal
conductivities of some common materials are listed. Air is a very
poor conductor of energy. Air is generally regarded as an
insulator. Water is a little bit better conductor. Metals
are generally very good conductors (cooking pans are often made of
stainless steel but have aluminum or copper bottoms to evenly spread
out heat when placed on a stove). Diamond has a very high
thermal conductivity. Diamonds are sometimes called "ice."
They feel cold when you touch them. The cold feeling is due to
the fact that they conduct energy very quickly away from your warm
fingers when you touch them.
The rate of energy transport also depends on
temperature
difference. If the object in the picture had been warm rather
than hot, less energy would flow or energy would flow at a slower into
the surrounding material.
Transport of energy by conduction is similar to the
transport of a strong smell throughout a classroom by diffusion.
Small eddies of wind in the classroom blow in random directions and
move smells throughout the room.. For our demonstration we used
curry powder.
The curry powder was actually
placed on a hot plate. With time the smell should have spread
throughout the room.
By the end of class some students
in the back of the room claimed
they could detect just the faintest hint of the curry smell.
Because
air has such a low thermal conductivity it is often used as an
insulator. It is important, however, to keep the air trapped in
small pockets or small volumes so that it isn't able to move and
transport energy by convection (we'll look at convection
shortly). Here are some examples of
insulators that use air:
Foam is filled with lots of small air bubbles
Thin insulating layer of air in a double
pane window
Hollow fibers (Hollofil) filled with air used in
sleeping
bags and
winter coats. Goose down works in a similar way.
Convection
was the next energy transport process we had a look at. We really
didn't have time to get into this very far in class on Tuesday.
Have a quick look through this material; we'll review it quickly in
class on Thursday.
Convection is energy transport by organized motion of the atoms or
molecules in a material. Rather
than moving about randomly, the atoms or molecules move as a
group. Convection works in liquids and gases but not
solids (the atoms or molecules are bound to each other and aren't able
to move around freely).
At Point 1 in the picture above a
thin layer of air
surrounding a hot object has
been
heated by conduction. Then at Point 2 a person (yes, that is a drawing
of a
person's head) is blowing the blob of warm air
off to the right. The warm air molecules are moving away at Point
3 from the
hot object together as a group (that's the organized part of the
motion). At Point 4 cooler air moves in and surrounds the hot
object and the whole process can repeat itself.
This is forced
convection. If you have a hot object in your hand you could just
hold onto it and let it cool by conduction. That might take a
while because air is a poor conductor. Or you could blow on the
hot object and force it to cool more quickly. If I had put a
small fan behind the curry powder demonstration y it would
probably have
spread the
smell faster and further out into the classroom.
A thin layer of air at Point 1 in
the figure above (lower
left) is
heated by conduction. Then because hot air is also
low density air, it actually isn't necessary to blow on the hot object,
the
warm air will rise by itself (Point 3). Energy is being
transported away
from the hot object into the cooler surrounding air. This is
called free convection and
represents another way of causing rising air motions in the
atmosphere. Cooler air moves in to take the place of
the rising air at Point 4 and the cycle repeats itself.
The example at upper right is also
free convection. Room temperature air in contact with a cold
object loses energy and becomes cold high density air. The
sinking
air motions that would be found around a cold object have the effect of
transporting energy from the room temperature surroundings to the
colder object.
In both examples of free convection, energy is being transported
from
hot toward cold.