Tuesday Aug. 31, 2010
click here to download today's notes in a more
printer friendly format
Two songs from Patty Griffin ("Stay
on
the
Ride", "Getting
Ready") before class today. I had planned to play a 3rd ("You'll
Remember") but didn't have time. They're all from her
"Children Running Through" CD.
There were a few questions about the Experiments.
Essentially all of the materials for Expt. #1 have been handed
out. The Expt. #1 reports aren't due until Tue., Sep. 21 but
there is no reason not to start the experiment now. When you are
done collecting your data you can return the materials (early) and pick
up a copy of the Supplementary Information handout that will help with
the analysis portion of your report. You can read a little bit
more about Expt. #1 here.
Experiment #2 uses the same graduated cylinders used in Expt.
#1. So the Expt. #2 materials won't be handed out until the week
of Sept. 21-24, i.e. after the Expt. #1 materials have been
returned.
There was a question last Thursday about why the earth's first
atmosphere was composed primarily of hydrogen (H) and helium
(He). The entire solar system formed from a large cloud of gases
called the solar nebula. The solar nebula itself was composed
mainly of H and He (98%). There were also small amounts (1.4%) of
H2O, CH4 (methane) and NH3 (ammmonia); minerals and rocks (0.4%), and
metals (0.2%) such as iron, nickel, and aluminum.
The inward pull of gravity caused the solar nebula to
contract. As it contracted it began to flatten and to spin.
The sun formed at the middle. The inner-most planets were close
enough to the sun that only the metals and minerals in the nebula were
able to condense. The earth's atmosphere was therefore made up of
the remaining gases (predominantly H and He but also small amounts of
H2O, CH4, and NH3).
We didn't have quite enough time last Thursday to finish p. 7 in
the photocopied ClassNotes that listed some of the important
characteristics of carbon monoxide. In particular we really
didn't discuss temperature inversions.
You'll find a typical winter morning temperature profile for Tucson at
the top of p. 9 in the ClassNotes.
There is very little vertical
mixing in a stable air layer.
When CO is emitted into the thin
stable layer (left figure
above), the CO
remains in the layer and doesn't mix with cleaner air above. CO
concentrations build.
In the afternoon, the ground warms, and the atmosphere becomes
more
unstable. CO emitted
into air at the surface mixes with cleaner air above. The CO
concentrations are effectively diluted.
Thunderstorms
contain strong up
(updraft) and down (downdraft) air motions. Thunderstorms are a
sure indication of unstable
atmospheric conditions. When the
downdraft winds hit the ground they spread out horizontally.
These surface winds can sometimes reach 100 MPH, stronger than many
tornadoes.
The
concentrations of several of the main pollutants are monitored
in
large cities in the US and around the world. Six pollutants are
listed below (p. 8 in the photocopied ClassNotes). In Tucson,
carbon monoxide, ozone, and particulate matter are of primary concern
and daily measurements are reported in the city newspaper. The
Air Quality Index value is reported instead of the actual
concentration. The AQI is the ratio of the measured to accepted
concentrations multiplied by 100%. Air becomes unhealthy when the
AQI value exceeds 100%.
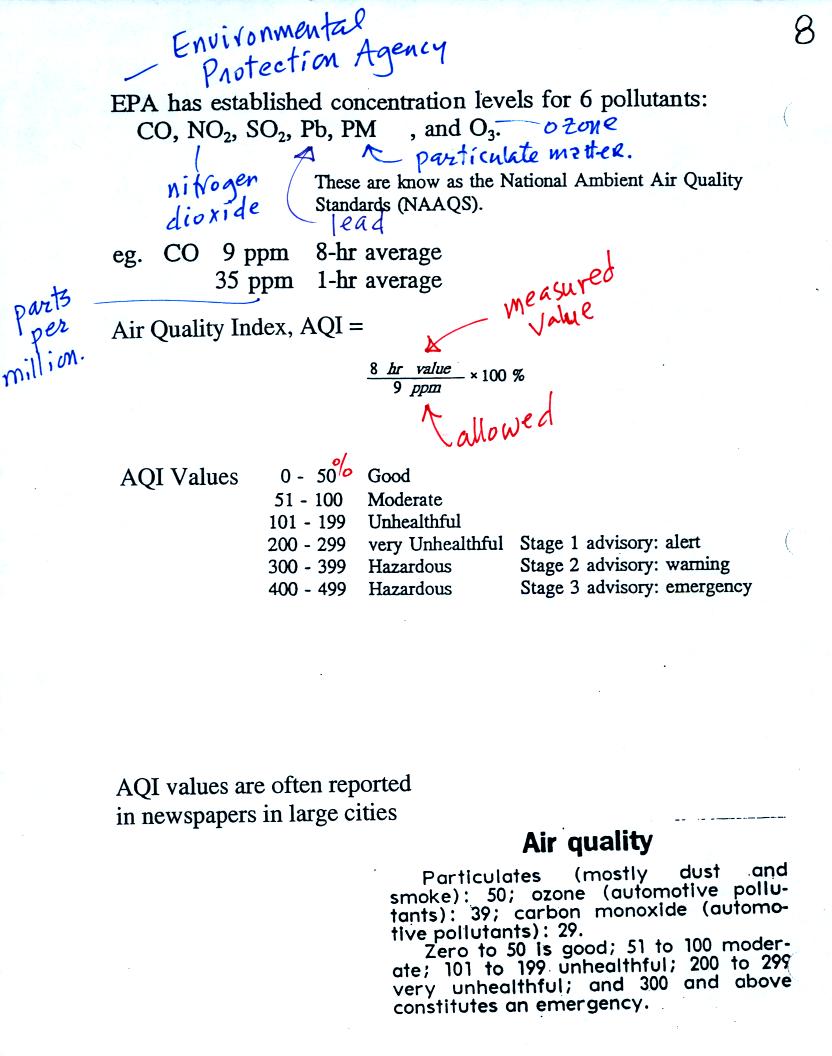
This is the page that I forgot to bring with me to class.
The atmospheric concentration of lead has decreased
significantly since the introduction of unleaded gasoline. PM
stands for particulate matter. These small particles are
invisible, remain suspended in the air, and may be made of harmful
materials. We'll talk about them in a little more detail on Thursday
For carbon monoxide,
concentrations up to 35 ppm (parts per million = 1 molecule of CO mixed
with 1 million molecules of air) for a 1 hour period and
9 ppm for an 8 hour period are allowed.
Here are a couple of example calculations (we did the first one in
class):
If the observed CO
concentration were 6 ppm averaged over an 8 hour period the AQI would
be
AQI = 100% x (6 ppm / 9ppm) = 67%
and the air quality would be
considered good.
What would the measured CO 8 hr average concentration be for an AQI
value of 33%?
Current Air Quality
Index values for
Tucson
are
available online.
Carbon monoxide is a serious
hazard indoors where is can build to much higher levels than would ever
be found outdoors. You may remember
having heard
about an incident at the beginning of the school year in 2007. Carbon
monoxide
from a malfunctioning hot water heater sickened 23 Virginia Tech
students in an apartment complex. The CO concentration is
thought to have reached 500 ppm. You can get an idea of what
kinds of health effects concentrations this high could cause from the
figure. on p. 9 in the photocopied ClassNotes.
The 400
ppm line in the ClassNotes approaches the level where CO would cause
coma and
death. At Virginia Tech several students were found unconscious
and one or two had stopped breathing but they were revived.
Carbon monoxide
alarms are relatively inexpensive (~$50) and readily available at a
hardware
store. They will monitor CO concentrations indoors and warn you
when
concentrations reach hazardous levels. Indoors CO is
produced by gas furnaces and water heaters that are
either operating improperly or aren't being adequately vented
to the outdoors. A few hundred people are killed indoors by
carbon
monoxide every
year in the United States. You can learn
more about carbon monoxide hazards and risk prevention at the Consumer Product
Safety Commission web page.
Here's a
figure I mentioned, but didn't cover in class. This is an
example of a habit I have of "beating some concepts to
death." The rather
busy picture below illustrates how small changes in how
air temperature changes with increasing altitude can determine whether
the atmosphere will be stable or unstable. Just for
the
purposes of illustration imagine riding a bicycle north from Swan and
River Rd up the hill to Swan and Sunrise (fhe figure shows an elevation
change of 1000 ft, it is actually quite a bit less than that).
At far left the air temperature goes from 47o F to 41o
F, a drop of 6o
F. This is a
fairly
rapid rate of decrease with increasing altitude and would make the
atmosphere
absolutely unstable.
The
atmosphere
wouldn't
remain
this
way.
Air
at
the
ground would rise, air higher up would sink, and
the
temperature profile would change (the rate of decrease with increasing
altitude would lessen). In some ways it would be like
trying to pour vinegar on top of oil in a glass. The lower
density oil would rise because it would "want" to float on top of the
higher density vinegar.
The next picture shows air temperature decreasing a little more slowly
with increasing altitude. This small change makes the atmosphere
conditionally unstable
(we won't go into what the conditions might
be). The
atmosphere is often in this state.
The atmosphere cools only 2o F in 1000 feet in the next
picture.
This creates
an absolutely stable
atmosphere. Air at the ground will remain at
the ground and won't rise and mix with air higher up. Compare
this with the glass containing vinegar and a layer of oil on top.
The two layers won't mix.
Air temperature in the last figure actually increases with increasing
altitude. This is a temperature
inversion and is very common on
winter mornings. The atmosphere is extremely stable under
these conditions.
Temperature inversions are something you can check out for yourself
later this semester. Head north on Swan
Rd. on your bicycle early some winter morning. You will pass
through
some pretty cold air as
you cross the Rillito River. By the time you get to Sunrise, the
air can be 10 to 15 degrees warmer and will seem balmy compared to the
cold
air at the bottom of the hill. If you're up for a real
hill-climbing challenge
continue north on Swan past Skyline. You'll find a short but very
steep section of road at the far north end of Swan.
As long as we're talking about bicycles and hills here's a picture
of my bicycle. I was in France in July 2009 trying to ride up
some
of the famous Tour de France mountain stages in the Alps. One of
the most famous is the Alpe d'Huez. That's my bicycle, a green
"Gilmour" (Andy Gilmour is a local bicycle builder) at the top of the
Alpe d'Huez.
The next picture shows the last 3 or 4 km of the road to the
summit of Mt. Ventoux, another famous climb in Provence. I was
there just a few weeks ago.
It was time, at this point, for the first of two class
demonstrations.
On Tuesday last week you were able to see a cloud form when moist
air
came
into contact with liquid nitrogen. You were also able to see a
cloud of photochemical smog in a demonstration later in today's
class. In both cases, the
droplets making up the clouds are probably too small to be seen by the
naked eye. You are able to see the clouds because the
cloud
droplets scatter light. The purpose of this demonstration was to
try to show you exactly what light scattering is.
In the first part of the demonstration a narrow beam of intense
red
laser light was shined from one side of the classroom to the
other.
The students couldn't see the laser beam because the light rays
weren't pointing straight at them. The instructor would have been
able
to see the beam if he had
walked to the wall and looked back along the beam of light (that
wouldn't have been a smart thing to do because the beam is strong
enough to damage his eyes).
Students were able to see a bright red spot where the laser beam struck
the wall.
This is because when the intense beam of laser light
hits the wall it
is scattered (splattered is a
more descriptive term). Weaker rays
of light are sent out in all directions. There is a ray of light
sent in the direction of every student in the class. They see the
light because they are looking back in the direction the ray came
from. It is safe to look at this light because the rays are
weaker than the initial beam.
Next we clapped some erasers together so that some small
particles of chalk dust fell into the laser beam.
Now instead of a single spot on the wall, students
saws lots of
points of light coming from different positions in a straight line
along the laser
beam. Each of these points of light was a particle of chalk, and
each piece of chalk dust was intercepting laser light and sending light
out in all directions. Each student saw a ray of light coming
from
each of the chalk particles.
We use chalk because it is white, it will scatter rather
than absorb visible light. What would you have seen if black
particles
of soot had been dropped into the laser beam?
In the last part of the demonstration we made a cloud by
pouring some
liquid nitrogen into a cup of water. The cloud droplets are much
smaller than the chalk particles but are much more numerous. They
made very good scatterers.
The laser light really lit up and
turned the small patches of
cloud
red. The cloud did a very good job of scattering laser light. So
much light was scattered
that the spot on the wall fluctuated in intensity (the spot dimmed when
lots of
light was being scattered, and brightened when not as much light was
scattered).
A comment that may not have been mentioned
in
class (if it was mentioned it certainly wasn't emphasized).
Air molecules are able
to scatter light too, just like cloud droplets. Air molecules are
much smaller than cloud droplets and don't scatter much light.
That's why you weren't able to see light being scattered by air before
we
put chalk particles or cloud droplets into the beam. Outdoors you
are able to see sunlight (much more intense than the laser beam used in
the class demonstration) scattered by air molecules. Sunlight is
white and is made up of violet, blue, green, yellow, orange, and red
light. Air molecules have an unusual property: they scatter the
shorter wavelengths (violet, blue, green) much more readily than the
longer wavelength colors in sunlight (yellow, orange, and red).
When you look away from the sun and look at the sky, the blue color
that you see are the shorter wavelengths in sunlight that are being
scattered by air molecules.
We'll come back to the topic of light scattering on Thursday. when we
cover particulate matter and its effect on visibility.
Next in our coverage of air pollutants is tropospheric ozone and
photochemical smog (aka Los Angeles type smog)
The figure above can be found on p.
14a in the photocopied ClassNotes. Ozone has a Dr. Jekyll (good)
and Mr. Hyde
(bad) personality. Ozone
in
the stratosphere (the ozone layer) is beneficial, it absorbs dangerous
high
energy ultraviolet light (which would otherwise reach the ground and
cause skin cancer, cataracts, and many other problems).
Ozone in the troposphere is bad, it is a
pollutant.
This is the stuff we will first be concerned with today.
Tropospheric
ozone is a key component of photochemical smog (also known as Los
Angeles-type smog)
We'll be making some photochemical smog as a
class
demonstration. This will require ozone (and a hydrocarbon of some
kind). We'll use the simple stratospheric recipe for making
ozone in the demonstration rather than the more complex tropospheric
process (4-step process shown below).
At the top of this figure you see
that a more complex
series
of
reactions is responsible for the production of tropospheric
ozone. The production of tropospheric
ozone begins with nitric
oxide
(NO). NO is produced when nitrogen and oxygen in air are heated
(in an
automobile engine for example) and react. The NO can then react
with oxygen to make nitrogen dioxide, the poisonous brown-colored
gas I decided not to make in class. Sunlight can dissociate
(split)
the nitrogen dioxide
molecule producing atomic oxygen (O) and NO. O and O2
react in a 4th step to make ozone (O3).
Because ozone
does not come directly from an automobile tailpipe or factory chimney,
but only shows up after a series of reactions, it is a secondary
pollutant. Nitric oxide would be the primary pollutant in
this example.
NO is produced early in the day (during the morning rush
hour).
The concentration of NO2
peaks
somewhat later. Because sunlight is needed in one of the
reactions and because peak sunlight normally occurs at noon, the
highest ozone concentrations are usually found in
the afternoon. Ozone concentrations are also usually higher in
the summer when the sunlight is most intense.
Once ozone is formed, the ozone can react with a hydrocarbon of some
kind to make a
product
gas. The ozone, hydrocarbon, and product gas are all invisible,
but the product gas sometimes condenses to make a visible smog
cloud or haze. The cloud is composed of very small droplets or
solid particles. They're too small to be seen but they are able
to scatter light - that's why you can see the cloud.
Here's a pictorial summary of the photochemical smog demonstration.
Once the cloud had formed we shined
the laser beam through the flask. Laser light wasn't visible to
the left or the right of the flask, only in the flask where smog
droplets were present and scattering laser light. The smog
droplets (and they may well be solid particles, I don't know) are very
small and even the weakest air current is able to keep them suspended.
Next we
moved on to the 3rd air pollutant that we will be discussing -
sulfur dioxide. Here's some basic information from the left hand
of p. 11 in the photocopied ClassNotes.
Sulfur dioxide is produced by the
combustion of sulfur
containing
fuels such as coal. Combustion of fuel also produces carbon
dioxide and carbon monoxide. People probably first became aware
of sulfur dioxide because it has an unpleasant smell. Carbon
dioxide and carbon monoxide are odorless. That is why sulfur
dioxide was the first pollutant people became aware of.
Volcanoes are a natural source of sulfur dioxide.
The
Great
London smog is still one of the two or three deadliest air pollution
events in
history. Because of a subsidence inversion the atmosphere was
stable and SO2
emitted into air
at ground level couldn't mix with cleaner air above (the surface
radiation inversions that we discussed at the beginning of class
usually last only a few hours, a subsidence inversion can last several
days). The SO2
concentration was able to build to dangerous levels.
4000 people
died during this 4 or 5 day period. As many as 8000
additional
people died in the following weeks and months.
Some
of the photographs below come from articles published in 2002 on the
50th anniversary of the event.
The sulfur dioxide didn't
kill people directly.
The SO2 aggravated
an existing
condition of some kind and hastened their
death.
The SO2 probably also made people susceptible
to bacterial
infections such as pneumonia.
This
link discusses the event and its health effects in more detail.
Note:
London type smog which contains sulfur dioxide and is
most
common
during the winter is very different from photochemical or Los Angeles
type smog. Los Angeles type smog contains ozone and is most
common in the summer.
Some other air pollution disasters also involved high SO2
concentrations.
One of the deadliest events in the US occurred in
1948 in Donora, Pennsylvania.
"This eerie photograph was taken at noon on Oct.
29, 1948 in Donora, PA as deadly smog enveloped the town. 20 people
were asphyxiated and more than 7,000 became seriously ill during this
horrible event."
from: http://oceanservice.noaa.gov/education/kits/pollution/02history.html
from: http://www.eoearth.org/article/Donora,_Pennsylvania
"When Smoke Ran Like
Water," a
book
about air pollution is among the books that you can check out, read,
and report on to fulfill part of the writing requirements in this class
(instead of doing an experiment report). The
author, Devra Davis, lived in Donora Pennsylvania at the time of the
1948 air pollution episode.