Tuesday Sept. 28, 2010
click here
to download today's notes in a more printer friendly format
The music before class today was from Brandi Carlile
("My Song", "Late Morning Lullaby", and "The Story" all from her The
Story CD)
I'll try to have the Expt. #1 reports graded by Thursday. You'll
then have ~2 weeks to revise your report.
The 1S1P reports that you're turning in today will be graded by ???
(the end of the semester for
sure). Actually we'll try to have them graded and returned to you
before the next report(s) are due.
Due next Tuesday, a Bonus 1S1P Assignment
that you will hear about in class today.
Coming on Thursday, a new Optional Assignment (that will be due next
Thu. - Oct. 6).
An ambitious schedule for today (order of presentation
subject to change)
1. Weather changes associated with the approach and passage of cold and
warm fronts
2. Locating fronts on surface weather maps
3. Deadly dangers associated with the Fall Equinox (might move to last
place)
4. Introduction to energy and energy transport
5. Relationship between energy added to a material and the temperature
change that results
6. An in-class measurement of the latent heat of vaporization of liquid
nitrogen
We didn't have time for the in-class experiment, we'll do that
on Thursday.
Here's a little
material that we didn't cover in class. I insert it only
because we covered it quickly in the MWF section and may have occasion
to refer to air masses by their different names.
An air
mass acquires its characteristics by remaining over a fairly
homogeneous surface (soil, ocean water, ice or snow) for some time it
will take on the characteristics
of the surface. Air masses are classified and named according to
their moisture and temperature
properties.
Continental polar air masses are cold and dry (the coldest,
driest, and densest of the 4 air masses). Martime polar air
masses
are somewhat warmer (ocean water doesn't get as cold as land masses)
and are
moister than cP air masses (though not as moist as mT air
masses). Continental polar air masses are warm and relatively dry
though usually not as dry as cP air masses. Martime tropical air
masses are the moistest air masses and can potentially produce the
greatest amounts of precipitation.
This next picture shows where these different types of air masses
originate.
We
spent a big part of the class today learning about some
of the weather conditions that precede and
follow passage of warm and cold fronts.
A crossectional view of a cold front is shown below:
Here are some of the specific weather changes
Weather
variable
|
Behind
|
Passing
|
Ahead
|
Temperature
|
cool, cold, colder*
|
|
warm
|
Dew Point
|
usually much drier*
|
|
may be moist (though that
is often
not the case here in the desert southwest)
|
Winds
|
northwest
|
gusty winds (dusty)
|
from the southwest
|
Clouds,
Weather
|
clearing
|
rain clouds, thunderstorms
in
narrow band along the front
(if the warm air mass is moist)
|
might see some high clouds
|
Pressure
|
rising
|
reaches a minimum
|
falling
|
* the coldest air might follow passage of a cold front by a day
or two. Nighttime temperatures often plummet in the cold dry air
behind a cold front. *Note the
green (cool), blue (cold), purple
(colder) sequence in the picture above behind the cold front.
A temperature drop is probably the most obvious change associated with
a cold front. Here in southern Arizona, gusty winds and a wind
shift are also often noticeable when a cold front passes.
The pressure changes that precede and follow a cold front are not
something we would observe or feel but are very useful when trying to
locate a front on a weather map.
In the next figure we started with
some weather data
plotted on a
surface map using the station model notation.
Before trying to locate a cold front, we needed to draw in a few
isobars and map out the pressure pattern. In some respects fronts
are like spokes on a wheel - they rotate counterclockwise around
centers of low pressure. It makes sense to first determine the
location of the low pressure center.
Isobars are drawn at 4 mb increments above and below a starting value
of 1000 mb. Some
of the allowed values are shown on the right side of the figure (992,
996, 1000, 1004, 1008 etc).
The highest pressure on the map is 1003.0 mb, the lowest is 994.9
mb. You must choose from the allowed list of isobar values and
pick only the values that fall between the high and low pressure values
on the map. Thus we have drawn in 996 mb and 1000 mb
isobars.
The next step was to try to locate the warm air mass in the
picture. Temperatures are in the 60s in the lower right portion
of the map; this area has been circled in red.
The cold front on the map seems to be properly postioned. The air
ahead of the front is warm, moist, has winds blowing from the S or SW,
and the pressure is falling. These are all things you would
expect to find ahead of a cold front. Clouds and a rain shower
were located right near the front which is
typically where they are found. The air behind the front is
colder, drier, winds are blowing from the
NW, and the pressure is falling. Note how the cold front is
positioned at the leading edge of the cold air mass, not necessarily in
front of the coldest air in the cold air mass.
Next we went through the same procedure with warm fronts.
Here's the crossectional view
Here ae the weather changes in advance of and following the frontal
passage.
Weather
Variable
|
Behind
(after)
|
Passing
|
Ahead
(before)
|
Temperature
|
warmer
|
|
cool
|
Dew point
|
may be moister
|
|
drier
|
Winds
|
from S or SW
|
|
from E or SE
|
Clouds, Weather
|
clearing
|
|
wide variety of clouds well
ahead of the front,
may be a wide variety of types of precipitation also.
|
Pressure
|
rising
|
minimum
|
falling
|
And here is the surface map analysis:
Note the extensive cloud coverage and precipitation found ahead of the
warm front. There is a pretty good temperature and dew point
difference on opposite sides of the warm front and a clear shift in
wind directions. Pressure is falling ahead of the warm front and
rising behind.
There was also pretty clear evidence of a cold front on this map.
The new Bonus
1S1P
Assignment will give you a chance to draw in some isobars and
locate a cold and warm front on your own. This Surface Weather
Map Analysis is due next Tuesday, Oct. 5.
For the next couple of weeks we
will be concerned with energy,
temperature, heat, energy transport, and
energy
balance between the earth, atmosphere, and space.
It is easy to
lose sight of the main concepts because there are so many
details. The following (found on pps 43&44 in the photocopied
Class Notes) is meant to introduce some of what we will be covering in
class. (the figures that follow are from a previous semester and
may differ somewhat from what we did in class)
Types of energy
We will learn the names of several different types or forms of
energy. Kinetic energy is energy of motion. Some examples (both large
and microscopic scale) are mentioned
and sketched above. This is a relatively easy to visualize and
understand form of energy.
Latent heat energy is perhaps the most underappreciated and most
confusing type of energy. The word latent refers to energy that is
hidden in water and water vapor. The hidden energy emerges when
water vapor condenses or water freezes.
Radiant energy is a very important form of energy that was for
some
reason left off the original list. Sunlight is an example of
radiant energy that we can see and feel (you feel warm when you stand
in sunlight). There are many types of radiant energy
that are invisible. Electromagnetic radiation is another name for
radiant energy.
Water vapor is a particularly
important form of
invisible
energy.
When water vapor condenses to produce the water droplets (or ice
crystals) in a
cloud, an enormous amount of latent heat energy is released into the
atmosphere.
It is hard to visualize or appreciate the amount of energy
released
into the
atmosphere during condensation. You can imagine the work that you
would do carrying a gallon of water
(8 pounds) from Tucson to the top of Mt. Lemmon. To
accomplish
the same thing Mother Nature must first evaporate the water and (if my
calculations are correct) that requires about 100 times the energy that
you would use to carry the 8 pounds of water to the summit of Mt.
Lemmon. And Mother Nature transports a lot more than just a
single gallon.
Energy transport
Four energy transport
processes are listed below.
By far the
most important process is energy transport in the form of
electromagnetic radiation (light is a common
form of electromagnetic radiation). This is the
only process that can transport energy through empty space.
Electromagnetic radiation travels both to the earth (from the sun) and
away from the earth into space. Electromagnetic radiation is also
responsible for about 80% of the
energy transported between the ground and atmosphere.
You might be
surprised to learn that latent heat is the second most important
transport process.
Rising parcels of warm air and sinking parcels of cold air are
examples of free convection. Because of convection you feel
colder or
a cold windy day than on a cold calm day.
Ocean currents are also an example of convection. Ocean currents
transport energy from the warm tropics to colder polar regions.
Remember that convection is one of the ways of causing rising air
motions in the atmosphere (convergence
into centers of low pressure, fronts, and orographic or topographic
lifting were the other ways).
Conduction is the least important energy transport at least in the
atmosphere. Air is such a poor conductor of energy that it is
generally considered to be an insulator.
Energy balance and the
atmospheric greenhouse effect
The next picture (the figure drawn in class has been split into three
parts for improved clarity) shows energy being transported from the sun
to
the earth in the form of electromagnetic radiation.
We are aware of this energy because
we can see it (sunlight
also contains invisible forms of light) and feel it. With all of
this energy arriving at and
being
absorbed by the earth, what keeps the earth from getting hotter and
hotter? The answer is that the earth also sends energy back into
space (the orange and pink arrows in the figure below)
This infrared light is an
invisible form of energy (it is weak enough that we
don't usually feel it either). A balance
between incoming and outgoing energy is achieved and the earth's annual
average temperature remains constant.
We will also look closely at energy transport between the earth's
surface and the atmosphere. This is where latent heat energy transport
and convection and conduction operate (they can't outside
the atmosphere into outer space).
That is also where the atmospheric
greenhouse operates. That will be a important goal -
to
better understand how the atmospheric greenhouse effect works.
The greenhouse effect is getting a
lot of "bad press". If the earth's atmosphere didn't contain
greenhouse gases and if there weren't a greenhouse effect, the global
annual average surface temperature would be about 0 F (scratch out -4 F
and put 0 F, it's easier to remember). With greenhouse gases this
average is about 60 F. This is the beneficial part of the
greenhouse effect.
The detrimental side is that atmospheric greenhouse gas concentrations
are increasing. This might enhance the greenhouse effect and
cause the earth to warm. While that doesn't necessarily sound bad
it could have many unpleasant side effects. That's a subject
while explore briefly later in the semester.
Now we start to get into some of the details.
When you
add energy to an object, the object will usually
warm
up (conversely when you take energy from an object the object will
cool). It is relatively easy to come up with an equation that
allows
you to figure out what the temperature change will be.
The temperature change will
first depend on
how much energy was added. This is a direct proportionality, so
delta E is in the numerator of the
equation (delta E and delta T are both positive when energy is added,
negative when energy is taken from something)
When you add equal amounts of energy to large and small pans
of water, the small pan will heat up more
quickly. The temperature change, delta T, will depend on the
amount of water, the mass. A small mass will mean a large delta
T, so mass should go
in the denominator of the equation.
Different materials
react differently when energy is added to them. A material with a
large specific heat will warm more slowly than a material with a small
specific heat. Specific heat has the same kind of effect on delta
T as
mass. Specific heat is sometimes called "thermal mass" or
"thermal capacity."
Here's an important example that will show the effect of specific
heat (middle of p. 45)
Equal
amounts of energy (1000 calories, note that calories are units of
energy) are added to
equal masses (500 grams) of water and soil. We use water and soil
in the
example because most of the earth's surface is either ocean or land.
Water has a higher specific heat than soil, it only warms up 2o
C.
The soil has a lower specific heat and warms up 10o C, 5
times more
than the water (there is a factor of 5 difference in the specific heats
of water and soil).
These different rates of warming of water and soil have
important effects on regional climate.
Oceans moderate the climate.
Cities near a large body
of water won't warm as much in the summer and won't cool as much during
the winter compared to a city that is surrounded by land.
The yearly high and low monthly average temperatures are shown at
two locations above. The city on the
coast has a 30o F annual range of temperature (range is the
difference between the summer and winter temperatures). The
city further
inland (assumed to be at the same latitude and altitude) has an annual
range of 60o F. Note that both cities have the same 60o
F annual
average temperature. We'll see a much more dramatic example of
the moderating effect of water on climate in a couple of weeks.
Here's
another situation where you
can take advantage of water's
high specific heat to moderate "micro climate."
Here are some tomatoes being
planted in early February in my
vegetable garden a winter or two ago. It still gets
plenty cold enough at night in February to kill tomatoes (the
brocolli
and
lettuce
in the background can handle a
light frost) so you have to protect them.
Here's one way of doing that.
You
can
surround
each
plant
with a "wall of water" - a tent
like arrangement that surrounds each plant. The cylinders are
filled with water and they take advantage of
the high specific
heat of water and won't cool as much as the air or soil would during a
cold
night.
Adding
energy to an object will usually cause it to warm. But there
is another possibility (bottom p. 45), the object could change
phase (change
from solid to liquid or
gas). Adding energy to ice might cause
the
ice to melt. Adding energy to water could cause it to
evaporate. The figure below is
a little more detailed version of what was drawn in class.
The equation at the bottom of the
figure above allows you to
calculate how much energy is required to melt ice or evaporate water or
sublimate dry ice. You multiply the mass by the latent heat, a
variable that depends on the particular material that is changing
phase.
The
fall (autumnal) equinox was on Wednesday (Thursday in
some locations) last week.
We would have had some kind of a celebration in NATS 101 if it hadn't
been quiz day.
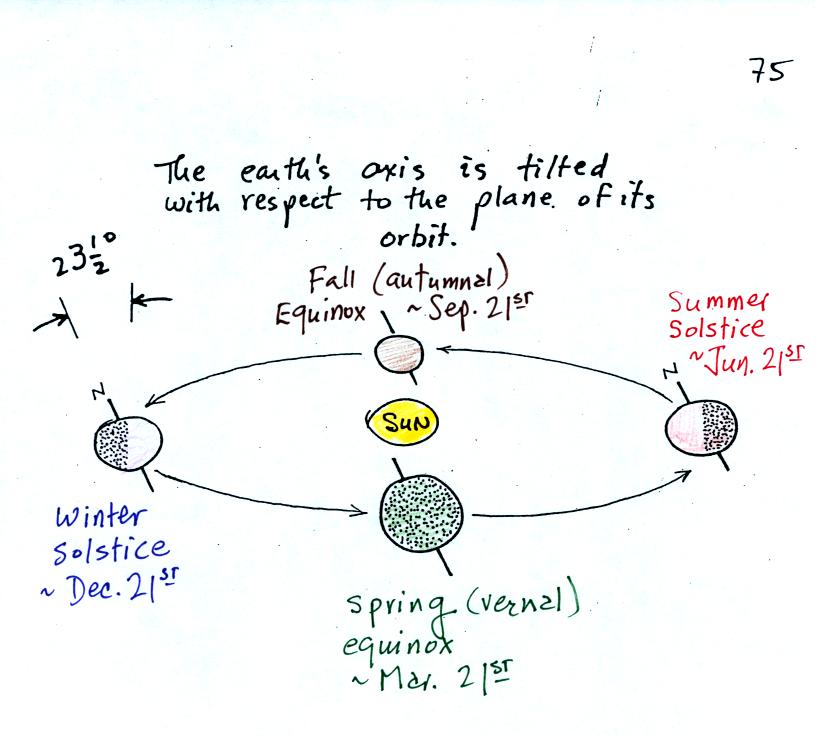
The figure above shows the earth orbiting the
sun. On
or
around Dec. 21st, the winter solstice, the north pole is tilted away
from the sun. Days are less than 12 hours long in the northern
hemisphere and the sun is low in the sky. Both factors reduce the
amount of sunlight energy reaching the ground. On June 21st, the
summer solstice, the north pole is tilted toward the sun. Days
are more than 12 hours long in the northern hemisphere and the sun is
high in the sky at noon. A lot more sunlight energy reaches the
ground; that's why it is summer.
The equinoxes are a time of transition. On the equinoxes, the day
and night are each 12 hours long everywhere on earth (except perhaps at
the poles). On the equinoxes, the sun rises exactly in the east
and
sets
exactly in the
west. The picture below shows the position of the sun at sunrise
(around 6:30 am on the spring and fall equinox in Tucson).
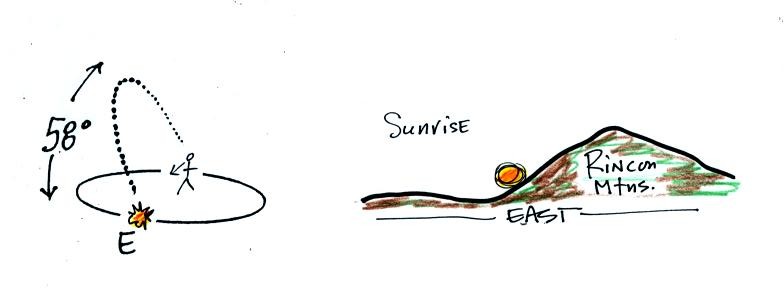
The figure at left traces out the path will follow in
the sky on the equinox. The sun rises in the east, moves to the
southern sky and begins to get higher in the sky
At noon you need to look about 60 degrees
above the
southern
horizon to see the sun. The sun is only 34.5 degrees above the
southern horizon on the winter solstice in Tucson and is
81.5 degrees
above the horizon, nearly overhead, at noon on the summer solstice.
On the equinoxes the sun sets exactly in the west at
about 6:30 pm.
The figure below shows you about what you would see if you
looked west on Speedway (from Treat Ave.) at sunset. In the
winter the sun will set south of west, in the summer north of west
(probably further south and north than shown here). On the
equinoxes the sun sets exactly in the west.
Several years ago I positioned myself in the median
near
the
intersecton of Treat and Speedway and pointed my camera west. I
took a multiple exposure photograph of the sun over a 2 hour period
that ended at sunset. I'll try to bring the slide photograph to
class one of these days.
If you aren't careful, you can get yourself seriously
injured,
even
killed,
on
or around the equinoxes.
I didn't have time
to show the
following figure in class.
June 21, the summer solstice, is the longest
day
of the
year (about 14 hours of daylight in Tucson). The days have slowly
been getting shorter since then. This will continue up until December
21,
the winter solstice, when there will be about 10 hours of
daylight. After that the days will start to lengthen as we make
our way back to
the summer solstice.
The length of the day changes most rapidly on the equinoxes.