Thursday Oct. 4, 2012
click here to
download today's notes in a more printer friendly format
"Dragonfly"
from
Ziggy
Marley and "Dar-Es-Salaam"
by
Ras
Nas before class this morning.
The Surface Weather Map Analysis that was turned in on Tuesday has
been graded and was returned in class today. Many of you will
find a little piece of paper attached to your work asking whether you
would prefer to earn extra credit or 1S1P points. Please return
those slips of paper to me. There were some very accurately drawn
maps turned in and also some that weren't quite as good. You'll
find a completed analysis here that you can
compare with your work.
Quiz #2 is one week from today and the Quiz
#2
Study
Guide is now available online. Note the two links to
sample questions (just questions, not answers) embedded in the
Study Guide.
I have finally set a due date for the 1S1P Assignment #2 reports -
Thursday Oct. 18.
The Upper Level Charts Optional Assignment was collected
today. You'll get the assignment back next Tuesday
with a Green Card attached if you scored 85% or above on the assignment.
One of my favorite graphics is a page from National Geographic
Magazine that lists some
of the limits
of
human
survival. I can't just scan the original and add it to the
notes without
violating copyright laws. But if you click on the link above
you'll find all of the same
information online in the form of a quiz.
I also showed a photograph of a nuclearn explosion which is a very
dramatic example of free convection. You'll find lots of
additional and much better photographs here.
You'll
also
find lots of videos on YouTube.
We spent a portion of the class period looking at latent
heat energy transport. This is the 3rd energy transport process
we have talked
about as we make our way from least important (in air that is
conduction) to most important (electromagnetic radiation).
If you had an object that you wanted to cool off quickly you could blow
on it. That might take a minute or two (maybe more). Or you
could stick it into some water, that would cool it
off pretty quickly because water will conduct energy more rapidly than
air. With a really hot object immersed in water,
you'd probably hear a brief sizzling sound, the sound
of boiling water. A lot of energy would be taken quickly from the
hot object and used to boil (evaporate) the water. The cooling in
this case takes only a few seconds.
Latent heat energy transport is sometimes a little hard to visualize
or understand because the energy is "hidden" in water vapor or water.
Latent heat energy transport is
associated with changes of
phase (solid to liquid, water to water vapor, that sort of thing) A
solid to liquid phase change is melting, liquid to gas is
evaporation, and sublimation is a solid to gas phase change. Dry
ice
is probably the best example of sublimation. When placed in a
warm room, dry ice turns directly from solid
carbon dioxide to gaseous carbon dioxide without melting first.
If you wash clothes and stick them outside on a cold (below freezing)
day they will eventually dry. The clothes would first freeze but
then
the ice would slowly sublimate away.
In
each case above energy must be added to the material changing
phase.
You can consciously add or supply the energy (such as when you put
water in a
pan and put the pan on a hot stove) or the phase change can occur
without you playing any role. In that case the needed energy will
be
taken from the surroundings.
Here's the simplest example I can think of.
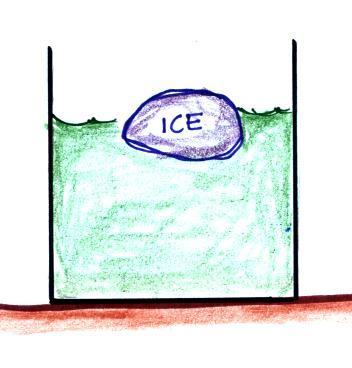
You put an ice cube
in a glass of warm water.
Energy will naturally flow from hot to cold; in this case from the
water (room temperature would be about 70 F) to the ice (32 F).
This transport of energy would occur via conduction..
Once the ice had absorbed enough energy it would melt.
Energy taken from the water would cause the water to
cool. The energy that needed to be added to the ice would be
taken
from the surroundings (the water) and would cause the
surroundings to cool.
Here's another example you should be very familiar with.
When you step out of the shower in
the morning you're covered with water. Some of the water
evaporates. It does so whether you want it to or not.
Evaporation requires energy and it gets that energy from your
body. Because your body is losing energy your body feels
cold.
The object of this figure is to
give you some appreciation for the
amount of energy involved in phase changes. A 240 pound man (I
usually use Tedy
Bruschi as an example) or
woman running at 20 MPH has just
enough
kinetic energy (if you could capture it) to
be able to melt an ordinary ice cube. It would take 8 people
running at 20 MPH to
evaporate the resulting ice water.
Phase changes can also go in the other direction.
You can consciously remove energy
from water vapor to make
it
condense. You take energy out of water to cause it to freeze (you
could put water in a
freezer; energy would flow from the relatively warm water to the
colder surroundings). If one of these phase
changes occurs, without you playing a role, energy will be released
into the surroundings (causing
the surroundings to warm). Note the orange energy arrows have
turned around and are pointing from the material toward the
surroundings. It's
kind of like a genie coming out of a magic lamp. One Tedy Bruschi
worth of kinetic energy is released when water freezes to make a single
ice cube. Many genies, many Tedy
Bruschis, are released when water vapor condenses.
This release of energy into the surroundings and the warming of the
surroundings is a little harder for us to appreciate because it never
really happens to us in a way that we can feel. Have
you ever stepped out of an air conditioned
building into warm moist air outdoors and had your glasses or
sunglasses "steam up"? That never happens to you (i.e. your body
doesn't steam up) because your body is too warm. However if it
did you would feel warm. It would be just the opposite of the
cold feeling when you step out of the shower or a pool and the water on
your body evaporates. You know how cold the evaporation can make
you feel, the same amount of condensation would produce a lot of
warming.
A can of cold drink will warm more
quickly in warm moist surroundings
than in warm dry surroundings. Equal amounts of heat will flow
from the warm air
into the cold cans in both cases. Condensation of water vapor is
an additional source of energy and will warm that can more
rapidly. I suspect that the condensation may actually be the
dominant process.
The foam "cozy", "koozie", or
whatever you want to call it, that you can put around a can of soda or
beer is designed to insulate the can from the warmer surroundings and
also to keep water vapor in the air from condensing onto the can.
This figure shows how energy can be
transported from one
location to another in the form of latent heat. The story starts
at left in the
tropics where there is often an abundance or surplus of sunlight
energy. Some of the incoming
sunlight
evaporates ocean water. The resulting water vapor moves somewhere
else and carries hidden latent heat energy with it. This hidden energy
reappears when something (air running into a mountain and rising,
expanding, and cooling) causes the water vapor to condense. The
condensation releases energy into the surrounding atmosphere.
This would warm the air.
Energy arriving in sunlight in the tropics has effectively been
transported to the atmosphere in a place like Tucson.
We're
ready
to
tackle
electromagnetic
radiation,
the most
important of the four energy transport processes (it's the most
important because it can carry energy through empty space).
First we need to review a
couple of rules concerning static electricity
and learn something about electric
field
arrows.
That's
all
we'll
have
time
for today.
The static electricity rules are found at the top of p. 59 in the
photocopied ClassNotes
Two electrical charges with the
same polarity push each other
apart. Opposite charges are attracted to each other.
There's a demonstration of these static electricity rules that I
would like to be able to show you. I haven't been able to get it
to work very well however. The demonstration involves a Van de
Graaff generator, something that produces a lot of electric charge and
high voltage. A wire connects the dome of the generator to a
small wand used to blow bubbles. Because of the connection to the
generator the bubbles are positively charged. As they drift
toward the dome of the generator the positive charge repels them and
they move away.
One of the big problems I've been having is with the bubbles.
They don't last very long and sink to quickly. While I haven't
been able to get the demonstration working very well I
did find a video
that you can watch and see how things should work.
Now the concept that we be using, electric field arrows. Electric
field arrows (or just the E field) show you the direction and
give you an idea of
the strength of the electrical force that would be exerted on a
positive charge
located at that point.
In this figure (p. 59 in the
ClassNotes) a positive charge has been placed at 3 locations around a
center charge. The electric field arrow shows the direction of
the force that would be exerted on each of the charges. The force
arrow is shown in blue. The forces range from weak to strong
depending on the distance between the two charges.
The E field arrows tell you what will happen to a + charge. but you can use the
arrows to determine what will happen to a - charge also.
For a negative charge the force will point in a direction opposite the
E field arrow.
Finally a couple of questions to test your understanding.
The first question comes from the Quiz #2 Study Guide.
What is the direction of the
electric field arrow at Point X halfway between a + and a -
charge?
The second question has two parts. First you need to determine
what polarity of charge must be on ground to cause the charges in the
figure below to move as they are doing. Then what direction does
the electric field arrow point at a location just above the ground
where the two charges are found.
Click here when
you think you
know the answers to these questions.
Now we'll use what we know about electric fields to start to
understand electromagnetic radiation.
An electric field
arrow
shows the
direction and
gives an idea
of the strength
of the
electrical force
that would be
exerted on a positive charge
You'll find most of the following on p. 60
in the photocopied ClassNotes.
We imagine turning on a source of
EM radiation and then
a
very short time
later we take a snapshot. In that time the EM radiation has
traveled to the right (at the speed of light). The EM radiation
is a wavy pattern of
electric and magnetic field arrows. We'll ignore the
magnetic
field lines. The E field lines sometimes point up, sometimes
down. The pattern of electric field arrows repeats itself.
Textbooks often represent EM
radiation with a wavy line like shown
above. They don't usually explain what the wavy line represents.
The wavy line just connects the
tips of a bunch of electric
field
arrows.
Note the + charge near the right
side of the picture. At the time
this
picture was taken the EM radiation exerts a fairly strong upward force
on
the
+
charge (we use the E field arrow at the location of the + charge to determine the direction
and strength of the force exerted on the + charge).
Th picture above was taken a short
time
after the first snapshot aftere the radiation
had
traveled a little further to the right. The EM radiation now
exerts a somewhat weaker downward force on the + charge.
A 3rd snapshot taken a short time
later. The +
charge is now being
pushed upward again.
A
movie
of
the +
charge, rather than just a series of snapshots, would show the
charge
bobbing up and down much like a swimmer in the
ocean would do as waves passed by.
The wavy pattern used to
depict EM radiation can be described spatially (what you would
see in a snapshot) in terms of its
wavelength,
the distance between identical points on the pattern.
Or you can
describe the radiation temporally
using the frequency of oscillation
(number of up and down cycles completed by an oscillating charge per
second). By temporally we mean you look at one particular fixed
point and look at how things change with time.
EM radiation can be created when
you cause a charge to move up and
down.
If you move a charge up and down slowly (upper left in the
figure above) you would produce long wavelength radiation that would
propagate out to the right at the speed of light. If you move the
charge up and down more rapidly you produce short wavelength radiation
that propagates at the same speed.
Once the EM radiation encounters the charges at the right side of
the
figure above the EM radiation causes those charges to oscillate up and
down. In the case of the long wavelength radiation the charge at
right oscillates slowly. This is low frequency and low energy
motion. The short wavelength causes the charge at right to
oscillate more rapidly - high frequency and high energy.
These three characteristics: long wavelength / low frequency / low
energy go
together. So do short wavelength / high frequency / high energy.
Note that the two different types of radiation both propagate at the
same speed.
The
following
figure
illustrates how energy can be
transported from one
place to another (even through empty space) in the form of
electromagnetic (EM) radiation.
You add energy when you cause an
electrical charge to move up and down
and create the EM radiation (top left).
In the middle
figure, the EM
radiation that is produced then travels out
to the
right (it could be through empty space or through something like the
atmosphere).
Once
the EM radiation encounters an electrical charge at another location
(bottom right),
the energy reappears as the radiation causes the charge to move.
Energy
has been transported from left to right.