Monday Feb. 19, 2007
There is a new 1S1P assignment. Read the instructions carefully
as there are two dates. There are also two worksheets that you
can complete with two of the topics for some extra credit. I
forgot to handout the worksheets in class, I'll do so in class on
Wednesday.
We
reviewed the section on energy transport by conduction and finished the
section on energy transport by convection that was started last Friday.
The following figures show some practical common applications of this
material.
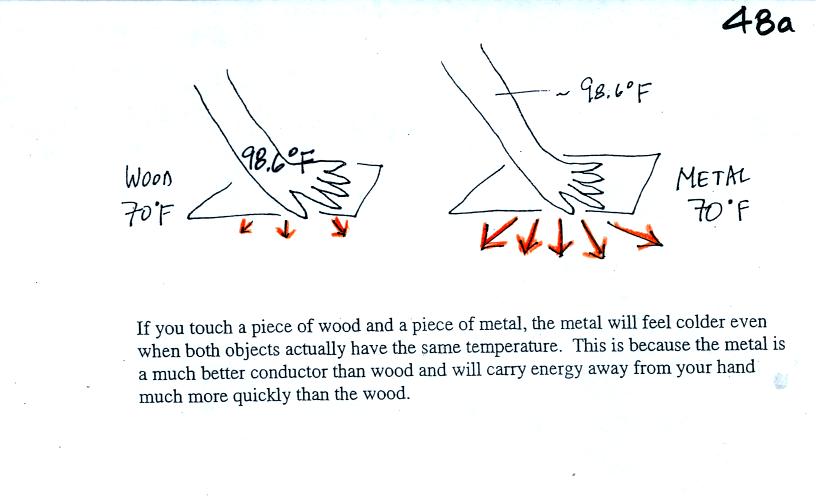
Metals are better conductors than wood. If you touch a
piece of
70 F metal it will feel colder than a piece of 70 F wood. A piece
of 70 F diamond would feel even colder because it is a better conductor
than metal. Our perception of cold is more an indication of how
quickly our hand is losing energy than a reliable measurement of
temperature.
Touching a piece of ice also feels cold even though ice is not an
especially good conductor. The cold feeling tells us that our
hand is losing a lot of energy. I this case the high rate of
energy loss is due to the large temperature difference between our
hand and the ice rather than just the thermal conductivity of the ice.
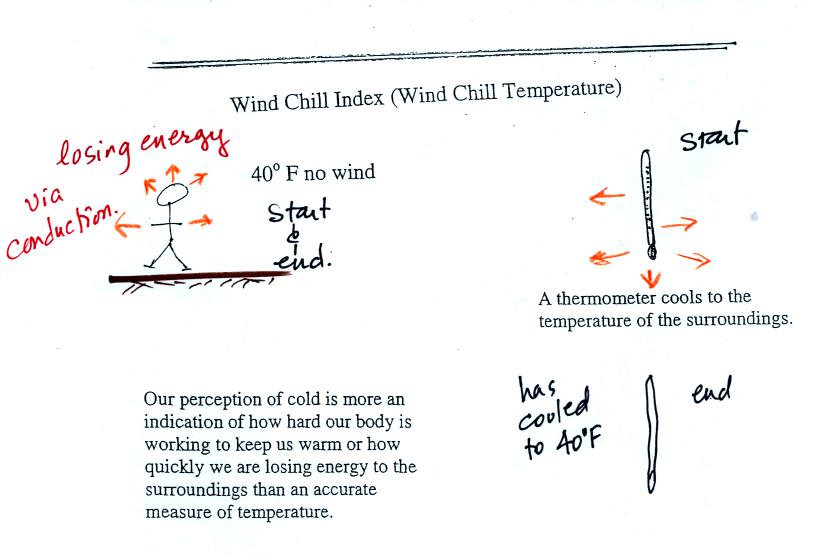
If you go outside on a 40 F day (calm winds) you will feel
cold; your
body is losing energy to the colder surroundings (by conduction
mainly). A thermometer
behaves differently. It actually cools to the temperature of the
surroundings. Once there it won't lose any additional energy.
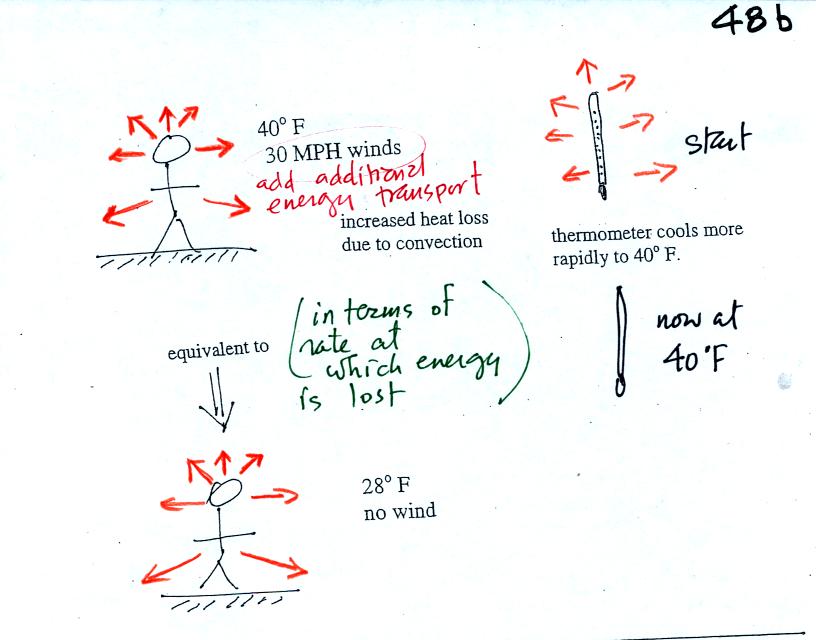
If you go outside on a 40 F day with 30 MPH winds your body
will lose
energy at a more rapid rate (because convection together with
conduction are transporting energy away from your body). It will
feel colder than a 40 F day
with calm winds. Actually, in terms of the rate at which your
body loses energy, the windy 40 F day would feel the same as a calm 28
F day. The combination 40 F and 30 MPH winds results in a wind
chill temperature of 28 F.
The thermometer will again cool to the
temperature of its surroundings, it will just cool more quickly on a
windy day. Once the thermometer reaches 40 F there won't be any
additional energy flow. The
thermometer would measure 40 F on both the calm and the windy day.
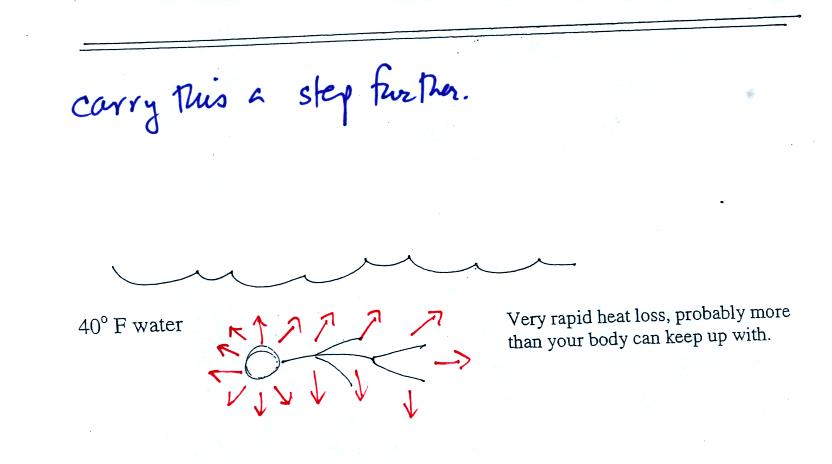
Water is a much better conductor than air. If you fall into 40 F
water your body will lose energy at a high enough rate that your
metabolism might not be able to keep up with it. Falling into 40
F water is a life-threatening situation.
Energy
transport in the form of latent heat is the second most important
energy transport process (second only to electromagnetic
radiation). It is a little tricky to see how the energy is
actually transported from one place to another. Before worrying
about that a little review is necessary.
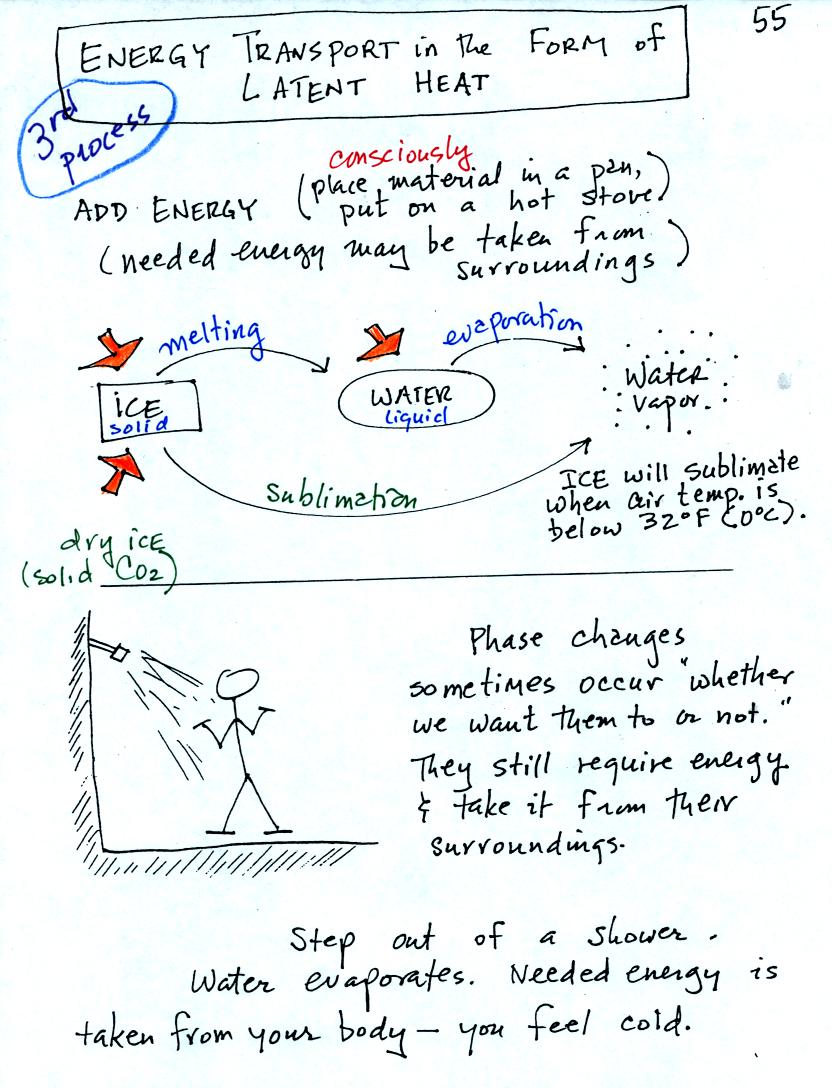
A solid to liquid phase change is melting, liquid to gas is
evaporation, and sublimation is a solid to gas phase change (dry ice
sublimates when placed in a warm room, it turns directly from solid
carbon dioxide to gaseous carbon dioxide).
In
each case energy must be added to the material changing phase.
You can consciously supply the energy (such as when you put water in a
pan and put the pan on a hot stove) or the needed energy will be
taken from the surroundings (from your body when you step out of a
shower in the morning).
Here's a school kids analogy:
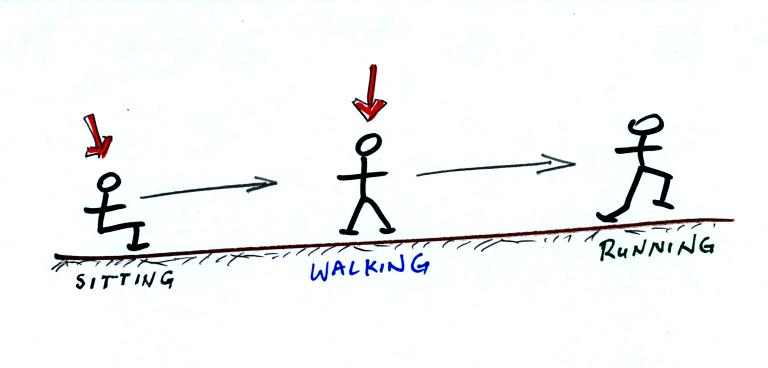
You need to give a kid some energy in order to get him or
her up and
walking around. Even more energy is needed to get the kid outside
running.
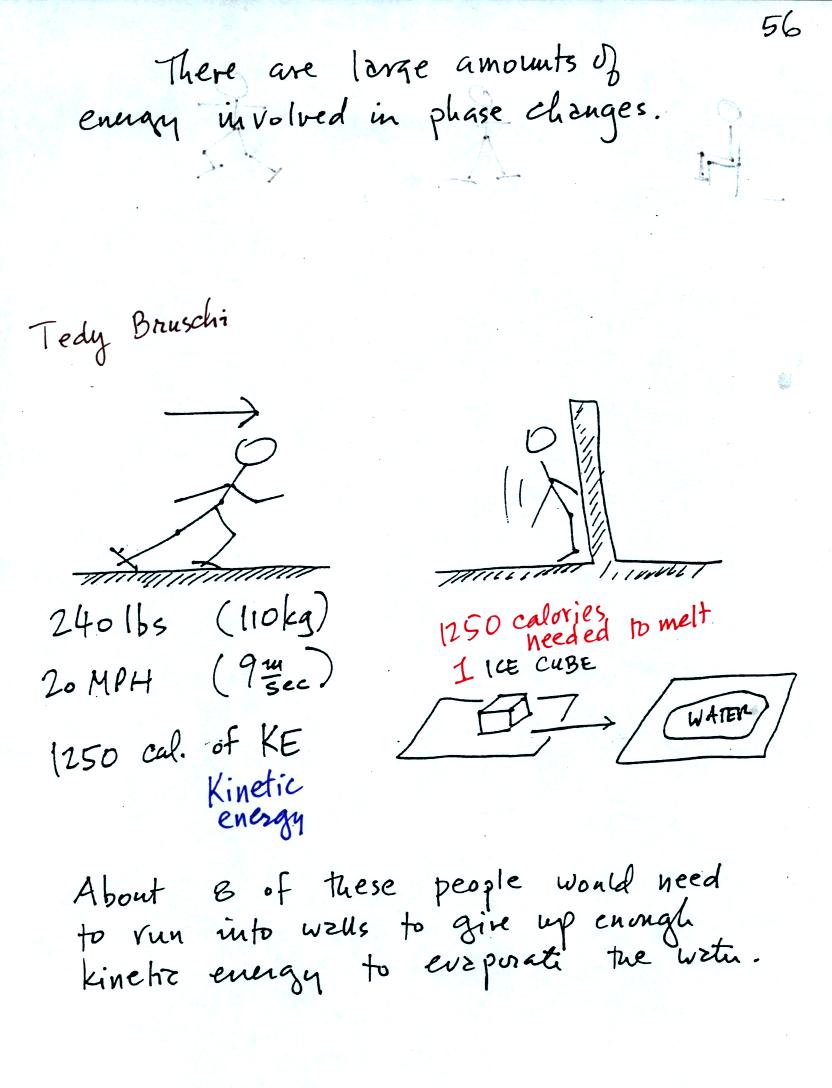
A 240 pound man (or woman) running at 20 MPH has just enough
kinetic energy (if you could somehow capture it) to
be able to melt an ordinary ice cube. It would take 8 people to
evaporate the resulting water.
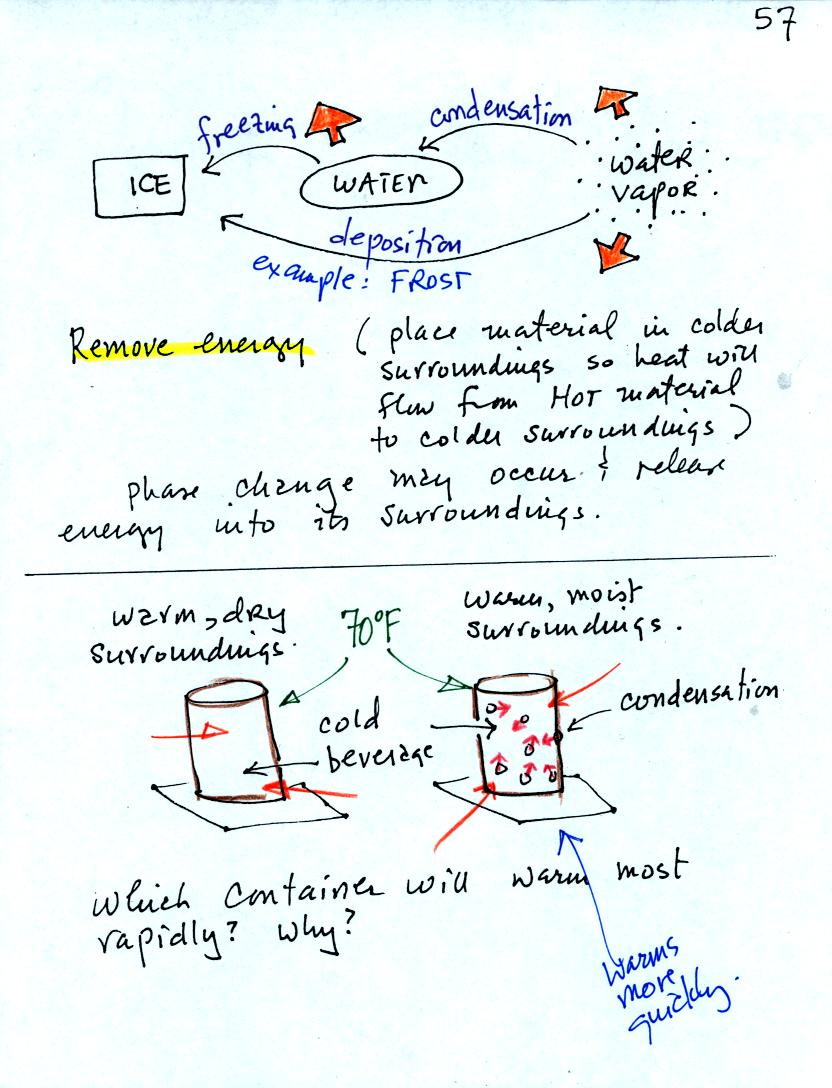
You can consciously remove energy from water vapor to make it
condense
or from water to cause it to free (you could put water in a freeze, a
cold "box"; energy would flow from the relatively warm water to the
colder surroundings). Or if one of these phase
changes occurs energy will be released into the surroundings (causing
the surroundings to warm). Note the orange energy arrows have
turned around and are pointing from the material toward the
surroundings.
A can of cold drink will warm more quickly in warm moist surroundings
than in warm dry surroundings. Heat will flow from the warm air
into the cold cans in both cases. Condensation of water vapor is
an additional source of energy and will warm that can more rapidly.
Here are the school kids again. They're out on the play ground
running around and you need to get them back inside the classroom and
sitting at their desks.
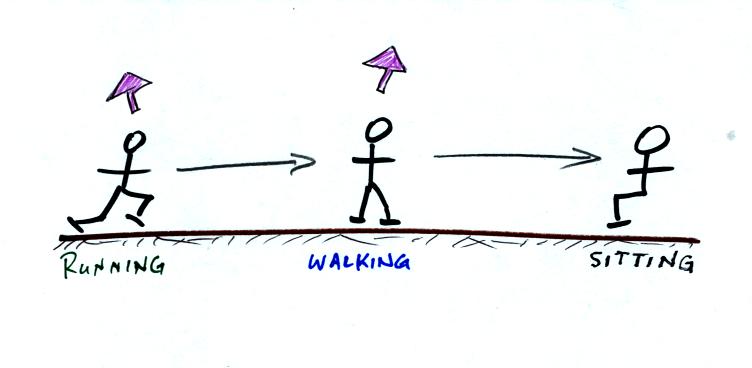
In this case you must take energy from the students to slow them down
and get them to walk instead of sun. Even more energy must be
removed in order to get them to sit still at their desks.
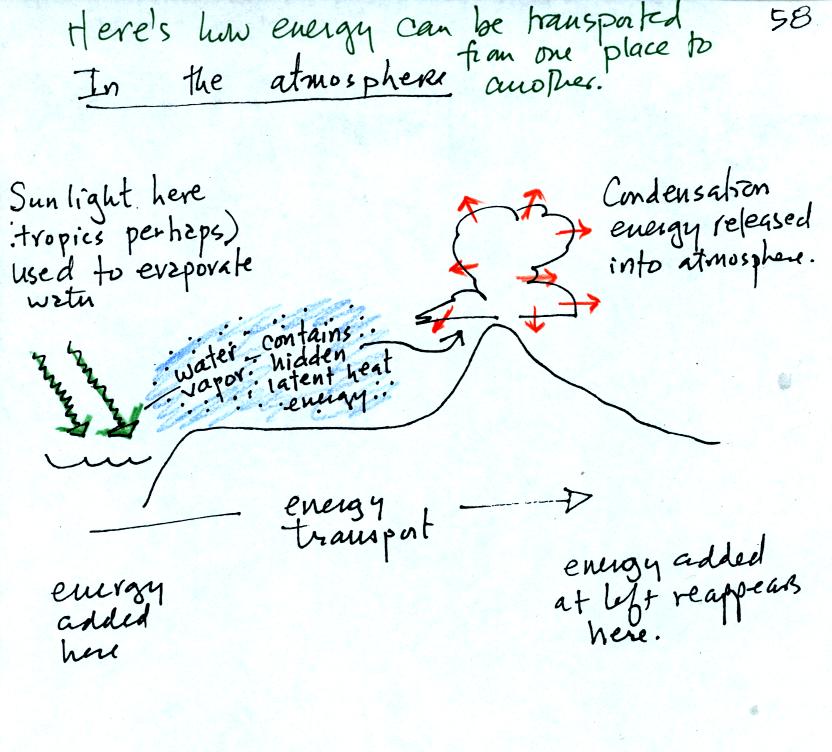
The story starts at left in the
tropics where there is often an abundance or surplus of energy;
sunlight
evaporates ocean water. The resulting water vapor moves somewhere
else and carries hidden latent heat energy. This hidden energy
reappears when something (air running into a mountain and rising,
expanding, and cooling) causes the water vapor to condense. The
condensation releases energy into the surrounding atmosphere.
Energy arriving in sunlight in the tropics has effectively been
transported to the atmosphere in Tucson.
Here are a
few sample energy transport questions.
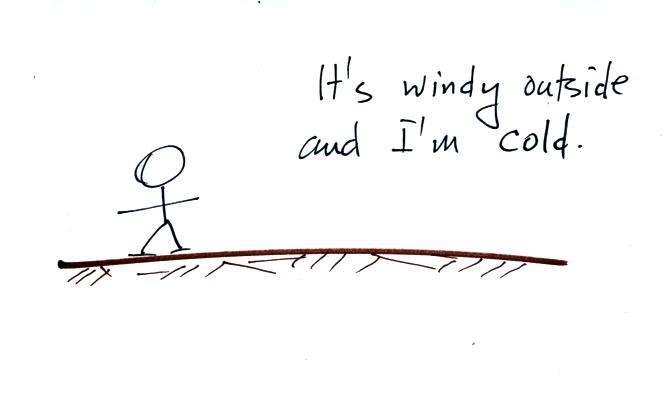
Question #1
What energy transport process is suggested?
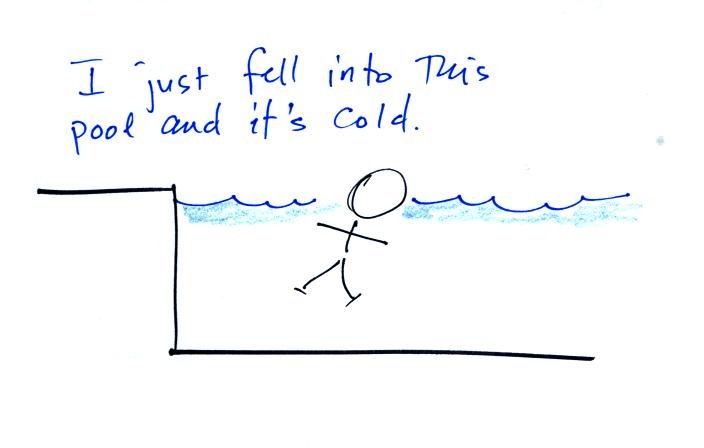
Question #2
What is the dominant energy transport process in this example?
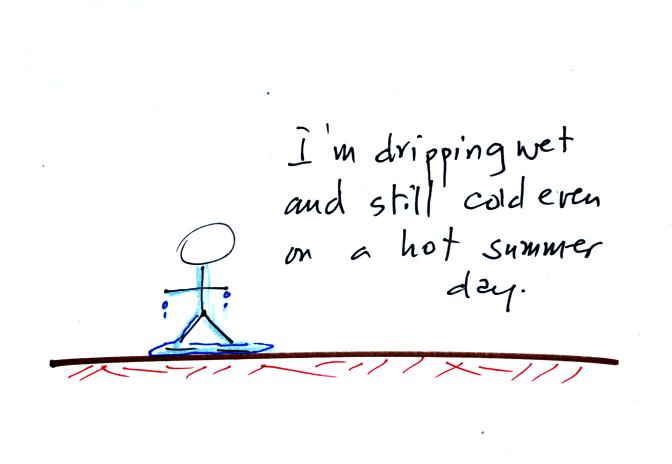
Question #3
How would you explain the feeling of cold in this case?
The next question wasn't asked in
class.
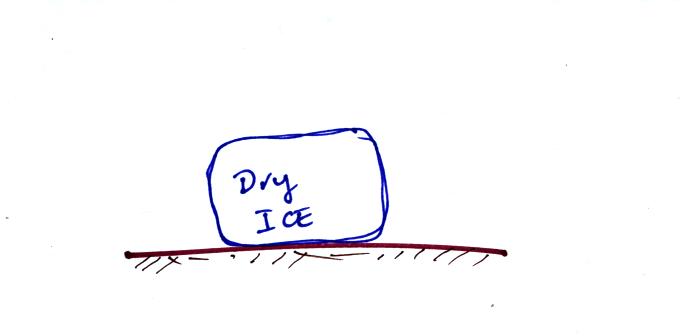
Question #4
A piece of dry ice is placed in a room.
Will energy flow from the
air TO the dry ice or FROM the dry ice to the air? What
does this flow of energy accomplish or do?
We'll
spend the next two or three class periods on electromagnetic
radiation. It is the most important energy transport process
because it can travel through empty space.
To really understand EM radiation you need to understand electric
fields. To understand electric fields we need to quickly review
static electricity.
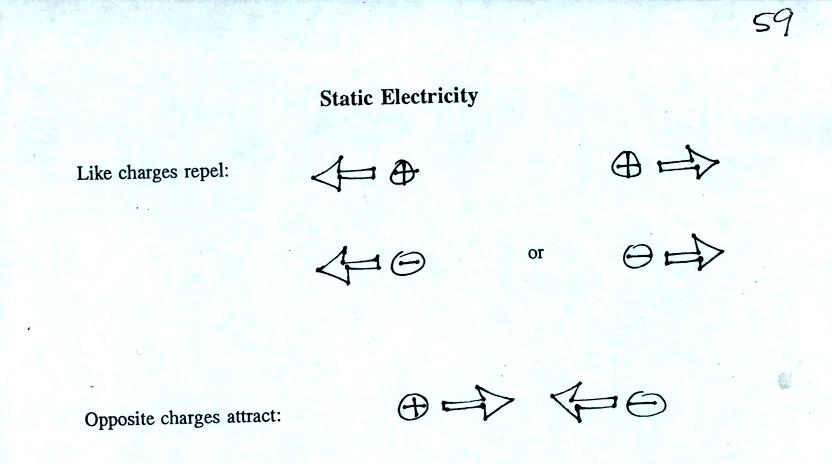
An electrical force is produced when two objects are placed
next to each other. The force can be repulsive or attractive.
We used a
sweater and two balloons to demonstrate the rules above.
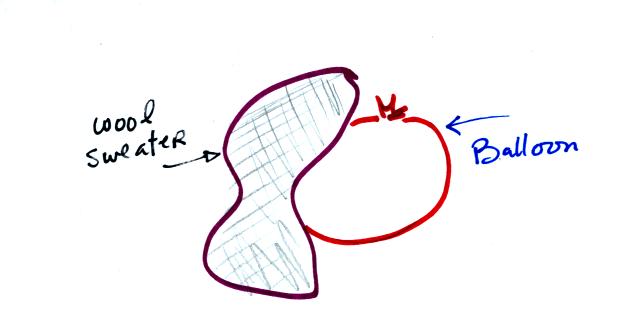
We rubbed two balloons with a sweater containing wool. The
balloons (and the sweater) became electrically charged (the balloons
had one polarity of charge, the sweater had the other).
We didn't know what charge
the balloons carried just that they both had the same charge.
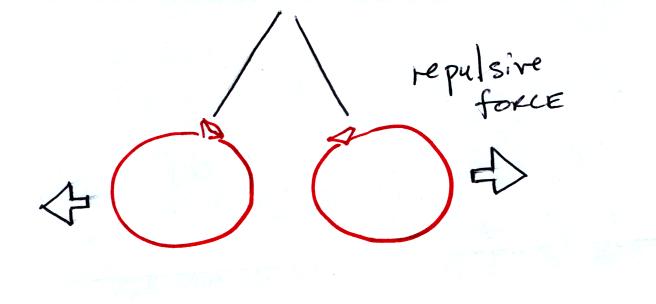
If you bring the balloons close to each other they are
pushed apart by
a repulsive electrical force.
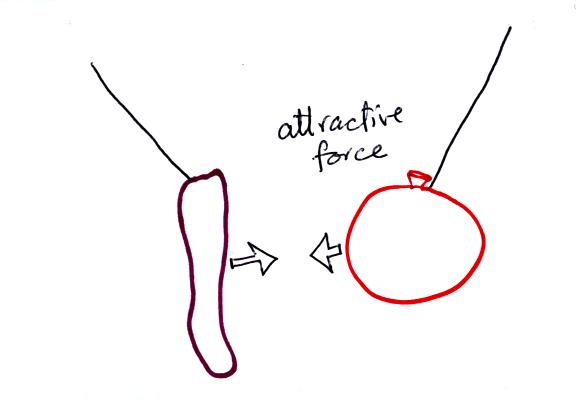
The sweater and the balloon carry opposite charges. IF
they are
brought together they experience an attractive electrical force.
The next
figure is from the bottom of p. 59 in the photocopied class notes.
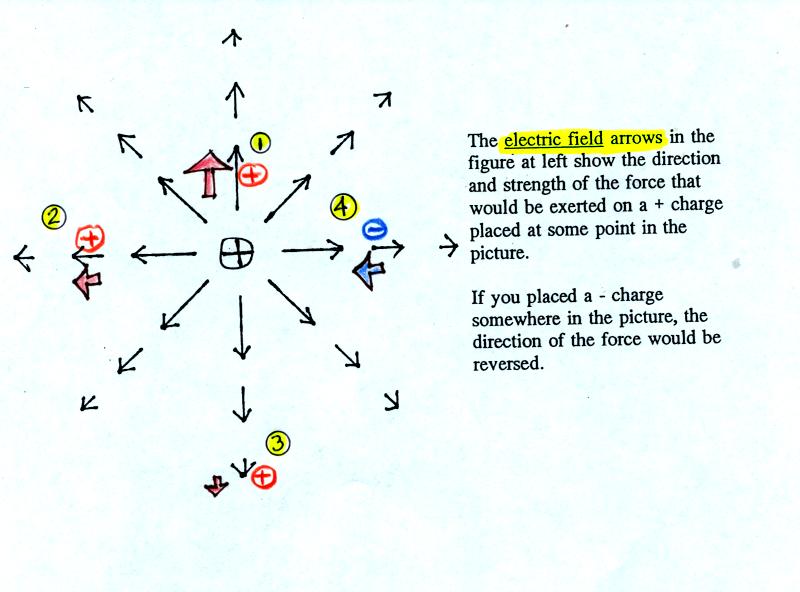
The balloons can help you understand
the picture above. Imagine placing one of the balloons at the
center of the picture and assume that it is positively charged.
The second balloon is placed at various positions (1, 2, and 3) around
the central balloon. The arrows in the picture are the electric
field. They give the direction and strength of the force that
would be exerted on the second positive charge. At Position 1,
for example a positively charged balloon would be pushed upward (by the
+
charge on the center balloon) with a strong
force. At Position 2 the force would point left but it isn't as
strong as at Pt. 1 because Position 2 is further from the center
charge. At Position 3 the charge is pushed downward with a
weak force.
You can also use the electric field arrows to figure out what would
happen to a negative charge. The direction of the force is
reversed. A negative charge would be pulled in toward the center
positive charge with moderate force.
Here are a couple of static electricity and electric field questions (these questions weren't asked in class).
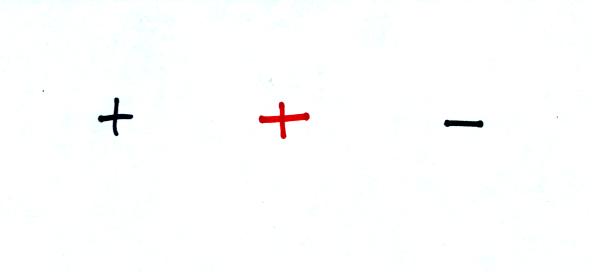
Question #5
A positive charge is placed halfway between another positive charge and
a negative charge. Will the center charge move RIGHT
LEFT or NOT MOVE at all?
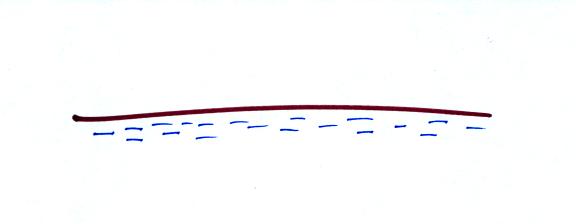
Question #6
The earth's surface is normally negatively charged. Will the
electric field in the air above the ground point UPWARD
or DOWNWARD?
Here are answers to
Questions 1-4.
Here are answers to
Questions 5&6.