Thursday Mar. 6, 2008
Quiz #2 Study Guide now available.
The Experiment #3 materials
were
distributed today. You will have another opportunity to check out
materials next Tuesday.
The Experiment #4 materials should also
become available next week.
Note: By the end of next
week you should either already have completed an experiment, should be
working on an experiment, or should be working on one of the other
options (book report, scientific
paper report).
We now
have most of the tools we will need to begin to study energy balance on
the earth. It will be a balance between incoming sunlight
energy and outgoing energy emitted by the earth. We will look at
the simplest case first, the earth without an atmosphere (or at least
an atmosphere without greenhouse gases) found on p. 68 in the
photocopied Classnotes.
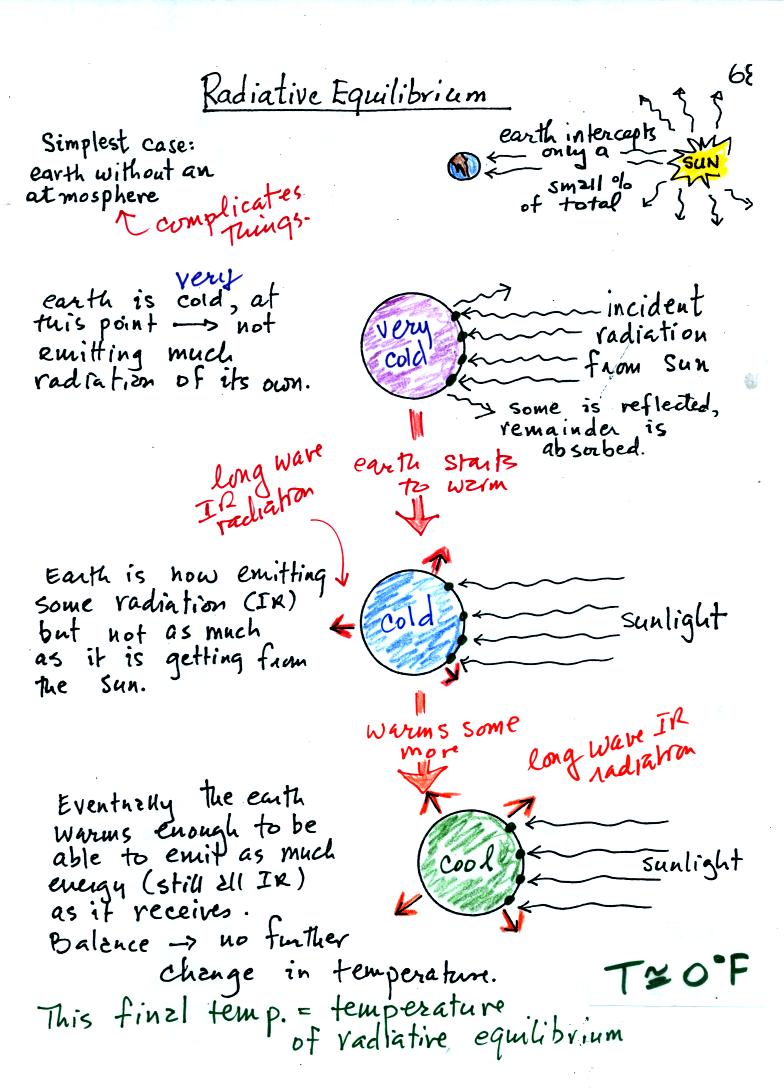
You might first wonder how, with the sun emitting so much
more
energy than the earth, it is possible for the earth (with a temperature
of around 300 K) to be in energy
balance with the sun (6000 K). The earth is located about 90
million miles
from the sun and therefore only absorbs a very small fraction of the
energy emitted by the sun.
To understand how energy balance occurs we start, in Step #1, by
imagining that the earth starts out very cold and is
not emitting
any EM radiation at all. It is absorbing sunlight however so it
will
begin to warm.
Once the earth starts to warm it will also begin to emit EM
radiation, though not as much as it is getting from the sun (the
slightly warmer earth in the middle picture is now colored blue).
Because the earth is still gaining more energy than it is losing the
earth will warm some more.
Eventually it will warm enough that the earth (now shaded green) will
emit the same amount
of energy (though not the same wavelength energy) as it absorbs from
the sun. This is radiative equilibrium, energy balance. The
temperature at
which this occurs is about 0 F.
That is called the temperature of radiative equilibrium. You
might remember this is the figure for global annual average surface
temperature on the earth without the greenhouse effect.
Before we
start to look at radiant energy balance on the earth we
need to learn about filters. The atmosphere will filter sunlight
as it
passes through the atmosphere toward the ground. The atmosphere
will
also filter IR radiation emitted by the earth as it trys to travel into
space.
We will first look at the effects simple blue, green, and red glass
filters have on visible light. This figure wasn't shown in class.

If you try to shine white light (a mixture of all the colors) through a
blue filter, only the blue light passes through. The filter
absorption curve shows 100% absorption at all but a narrow range of
wavelengths that correspond to blue light. Similarly the green
and red filters only let through green and red light.
The following figure is a simplified easier to remember
representation of the
filtering effect of
the atmosphere on UV, VIS, and IR light (found on p. 69 in the
photocopied notes). The
figure below was redrawn after class for improved clarity.
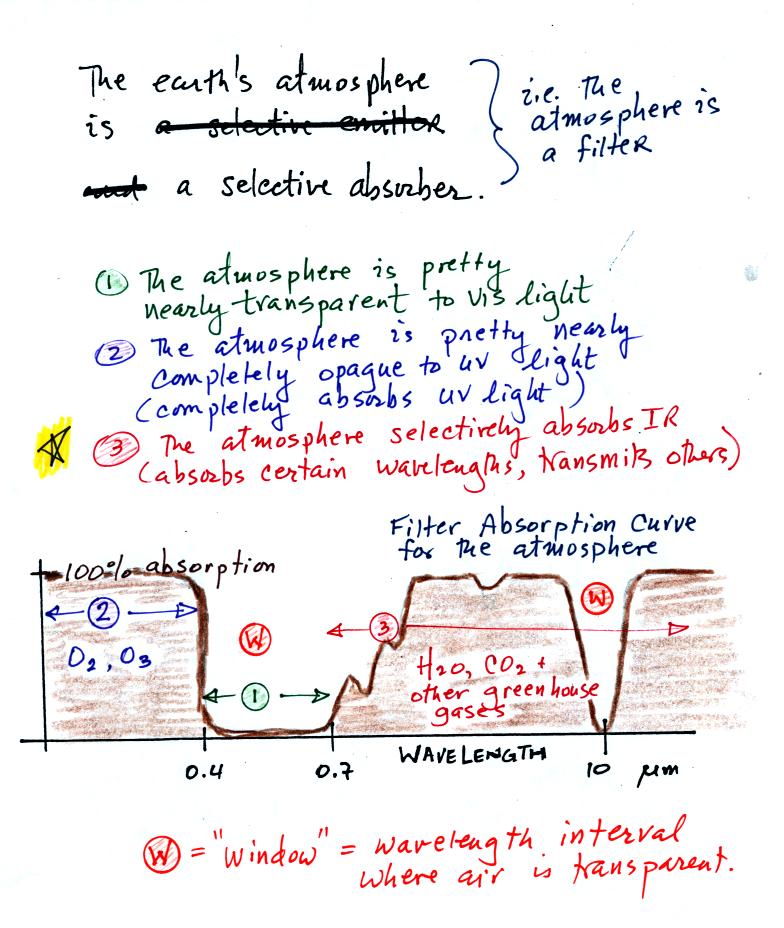
You can use your own eyes to tell you what the filtering
effect of the
atmosphere is on visible light. Air is clear, it is
transparent. The atmosphere transmits visible light.
In our simplified representation oxygen and ozone make the atmosphere a
pretty good absorber of UV light.
Greenhouse gases make the
atmosphere a
selective absorber of IR light - it absorbs certain IR wavelengths and
transmits others. It is the atmosphere's ability to absorb (and
also emit) certain wavelengths of infrared light that produces the
greenhouse effect and warms the surface of the earth.
Note "The atmospheric window"
centered at 10 micrometers. Light emitted by the earth at this
wavelength will pass through the atmosphere. Another transparent
region, another window, is found in the visible part of the spectrum.
You'll find a more realistic picture of the atmospheric absorption
curve on p. 70 in the photocopied Classnotes, but the simplified
version above will work fine for our needs.
Here's the
outer space view of radiative equilibrium on the earth without an
atmosphere. The important thing to note is that the earth is
absorbing and emitting the same amount of energy (4 arrows absorbed
balanced by 4 arrows emitted).
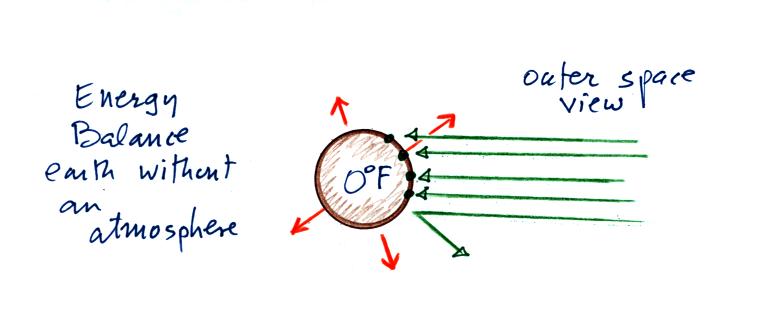
We will be moving from an outer space vantage point of
radiative equilibrium (above) to the earth's
surface (below).
Don't let the fact that there are
4 arrows are being absorbed and
emitted in the top figure and
2 arrows absorbed and emitted in the bottom figure
bother you
We'll be adding a lot more arrows to the bottom figure
It would get too complicated if we had more than 2 arrows of incoming
sunlight.
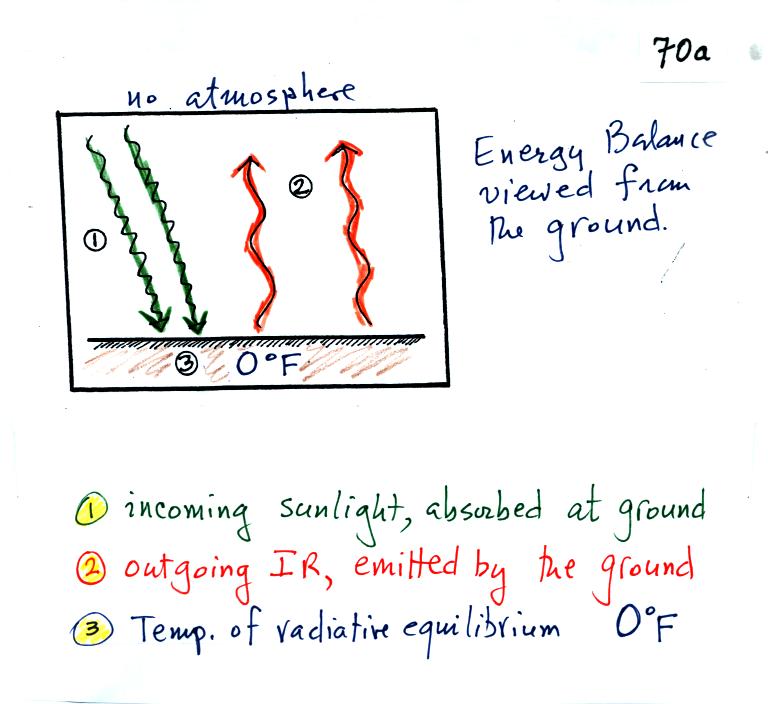
The next
step is to add the atmosphere.
We will study a simplified version of radiative equilibrium just so you
can identify and understand the various parts of the picture.
Keep an eye out for the greenhouse effect. We will look at a more
realistic version later.
Here's the figure that we ended up with in class
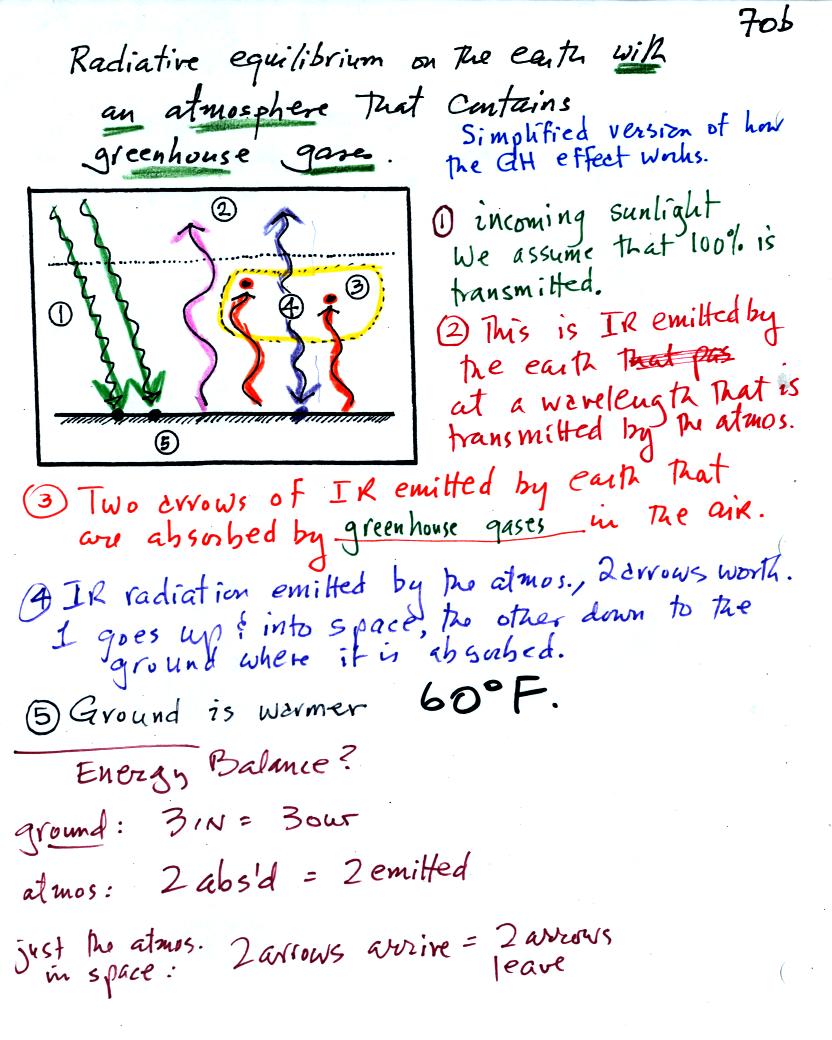
It would be hard to sort through all of this if you weren't in class
(and maybe even if you were) to see how it developed. So below we
will go through it again step by step (which you are free to skip over
if you wish).

The figure shows two rays of incoming sunlight that
pass through the atmosphere, reach the ground, and are absorbed.
100% of the incoming sunlight is transmitted by the atmosphere (this is
not a very realistic assumption).
The ground is emitting 3 rays of IR radiation.
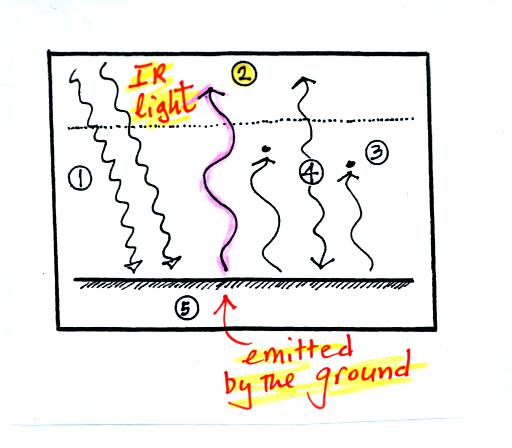
One of these is emitted by the ground at a wavelength that is
NOT absorbed by greenhouse gases in the atmosphere. This
radiation passes through the atmosphere and goes out into space.
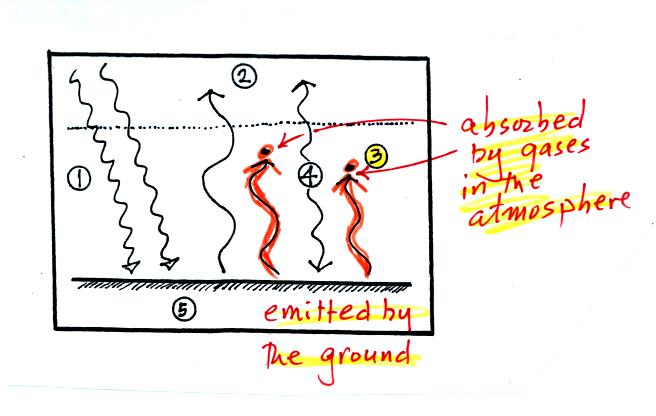
The other 2 units of IR radiation emitted by the ground are
absorbed by
greenhouse gases is the atmosphere.

The atmosphere is absorbing
2 units of radiation.
In order to be in radiative equilibrium,the atmosphere must also emit 2
units of radiation. 1
unit of IR radiation is sent upward into space, 1 unit is sent downward
to the ground where it is absorbed.
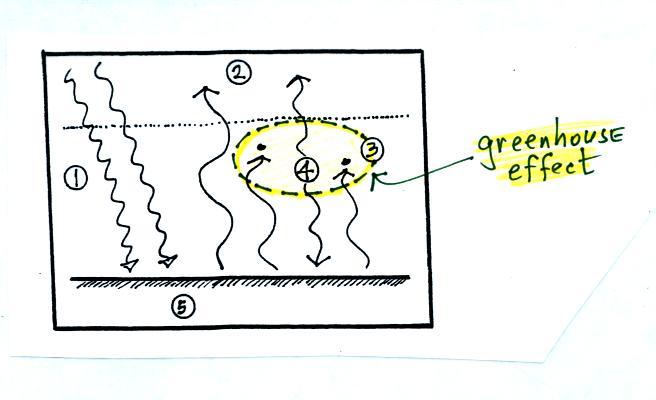
The greenhouse effect is found in this absorption and emission
of IR radiation by the atmosphere. We tried to put into words
what is illustrated above:
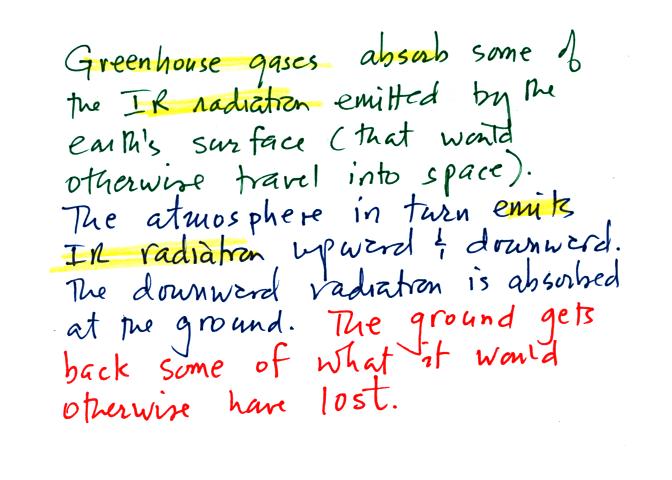
Before we go any further we will check to be sure that
every part
of this picture is in energy balance.
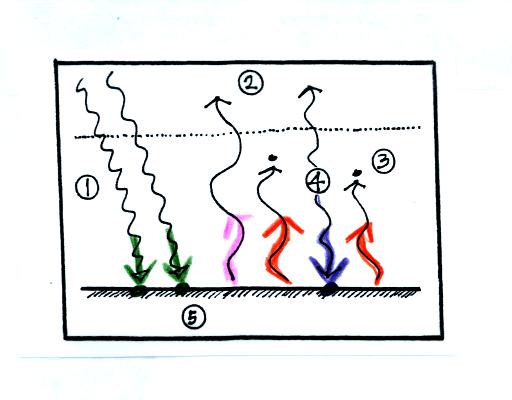
The ground is absorbing 3 units of energy and emitting
3
units of energy
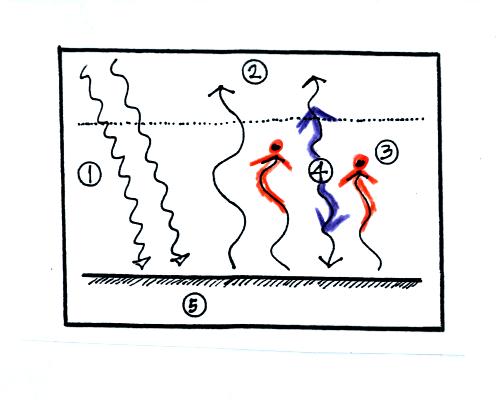
The atmosphere is absorbing 2 units of energy and emitting 2
units of
energy
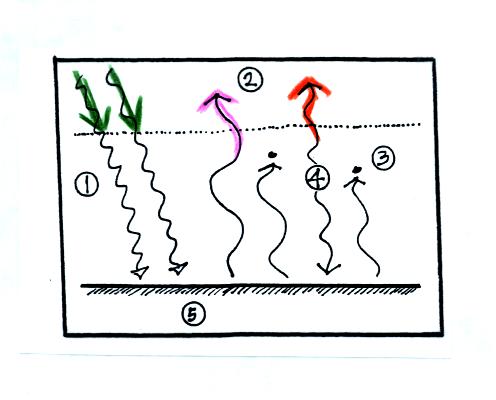
2 units of energy arrive at the earth from outer space, 2 units
of
energy leave the earth and head back out into space.
The
greenhouse effect makes the earth's surface warmer than it would be
otherwise.
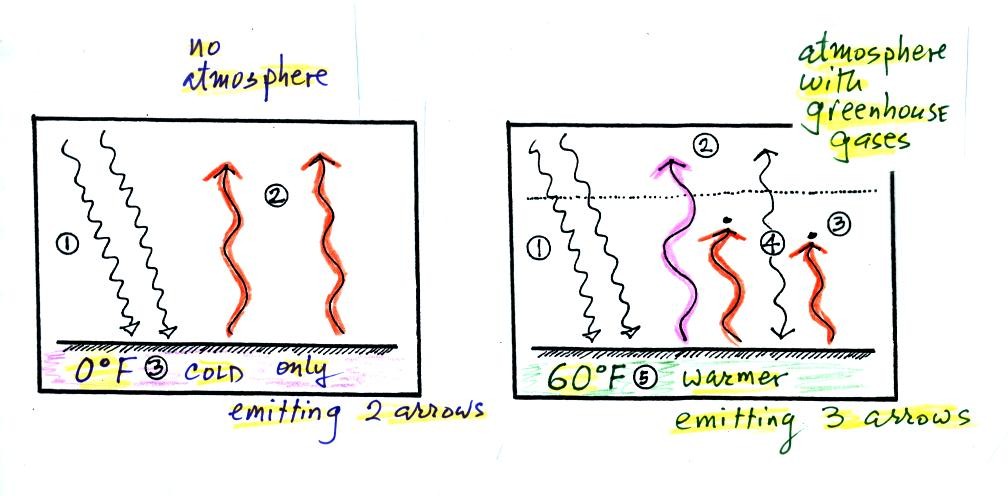
Energy balance with (right) and without (left) the
greenhouse
effect. At left the ground is emitting 2 units of energy, at
right the ground is emitting 3 units. Remember that the amount of
energy emitted by something depends on temperature. Warm ground
will emit more energy than colder ground.
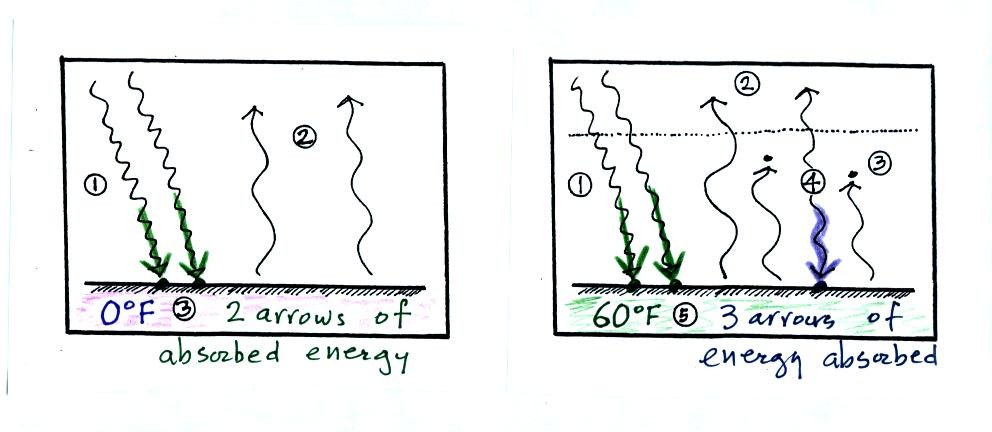
Here's another explanation. At left the ground
is getting 2 units of energy. At right it is getting three, the
extra one is coming from the atmosphere. Doesn't it make sense
that ground that absorbs 3 units of energy will be warmer than ground
that is only absorbing 2.
Next
we will look at how realistic our simplifying assumptions are

In our simplified version of the greenhouse
effect we
assumed that
100%
of the sunlight arriving at the top of the atmosphere passes through
the atmosphere and gets absorbed by the ground. The bottom figure
above shows that in reality only about 50% of the incoming sunlight
gets absorbed at the ground.
About 20% of the incoming sunlight is absorbed by gases in the
atmosphere. Sunlight is a
mixture of UV, VIS, and IR light.
Ozone and oxygen will absorb a lot of the UV (though there isn't much
UV in sunlight) and greenhouse gases will absorb some of the IR
radiation in sunlight (IR light accounts for about half of the light in
sunlight).
The remaining 30% of the incoming sunlight is reflected back into space
(by the ground, clouds, even air molecules).
Now we
will look at our simplified version of radiative equilibrium and a more
realistic picture of the earth's energy budget.
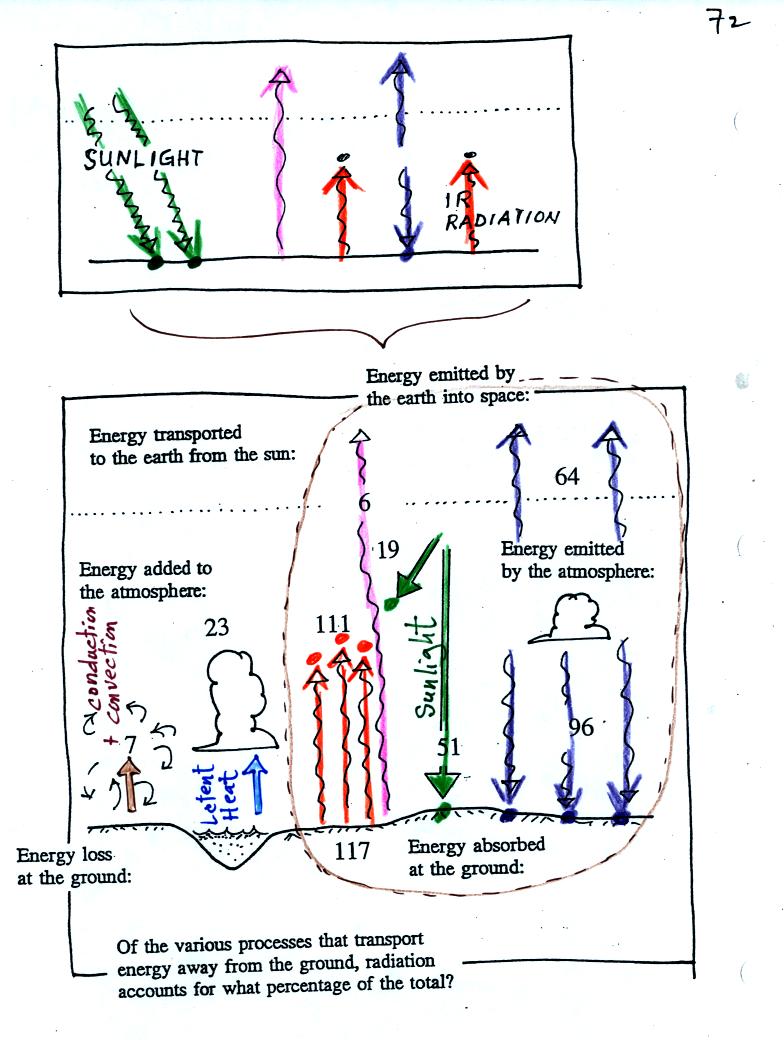
The lower part of the figure is pretty complicated. It would be
difficult to start with this figure and find the greenhouse effect in
it. However if you understand the upper figure, you should be
able to find and understand the corresponding part in the lower figure.
In the top figure you should recognize the incoming sunlight (green),
IR emitted by the ground that passes through the atmosphere (pink), IR
radiation emitted by the ground that is absorbed by greenhouse gases in
the atmosphere (orange) and IR radiation emitted by the atmosphere
(dark blue). Using the colors you can find each of these parts of
the
energy budget in the bottom figure. Notice that conduction,
convection, and latent heat energy transport are needed to bring the
overall energy budget into balance. Click here to see a more detailed
check to be sure everything is in energy balance.
We took a short detour at this
point to look at Archimedes law (see pps 53a & 53b in the
photocopied Classnotes) and to see a colorful demonstration.
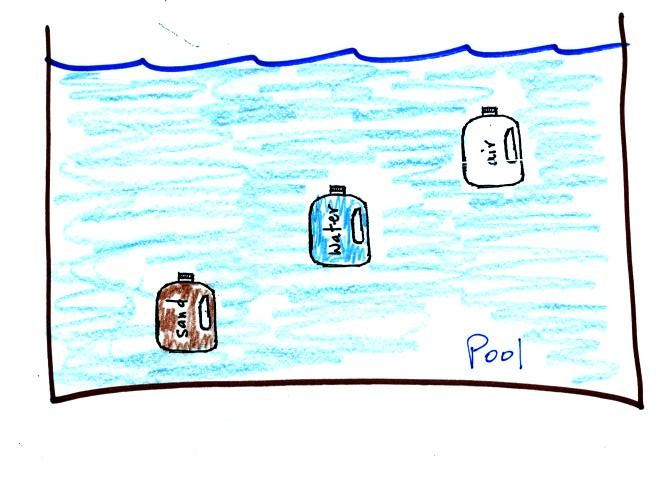
A gallon of water weighs about 8 pounds, that's pretty
heavy. If you jump into a pool with a gallon bottle of water, the
bottle becomes weightless. Archimedes law explains why this is so.
Archimedes law says that an object immersed in a fluid (gas or liquid)
will experience an upward bouyant force equal to the weight of the
fluid displaced.
The gallon water bottle will displace 1 gallon of pool water. The
8 pound weight (gravity) will be balanced by an 8 pound upward bouyant
force.
If you filled the bottle with air (which has practically zero weight),
the bottle will float. The 8 pound upward bouyant force is still
there because the bottle still displaces a gallon of pool water.
But the downward force (weight of the air) is gone.
If you fill the bottle with sand, the bottle will now weigh about 12
pounds. That's more than the 8 pound upward bouyant force, so the
bottle will sink.
Basically its a question of how the density of the material in the
bottle compares to the density of the water outside. Objects
denser than water will sink, objects less dense than water will float.
This can be applied to the atmosphere
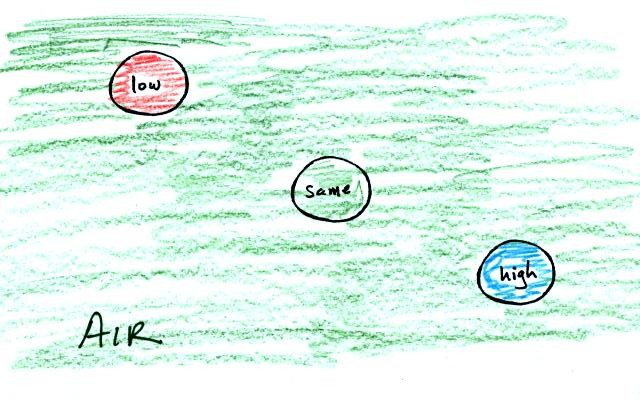
Warm low density air rises, cold high density air sinks.
You can also apply this to people
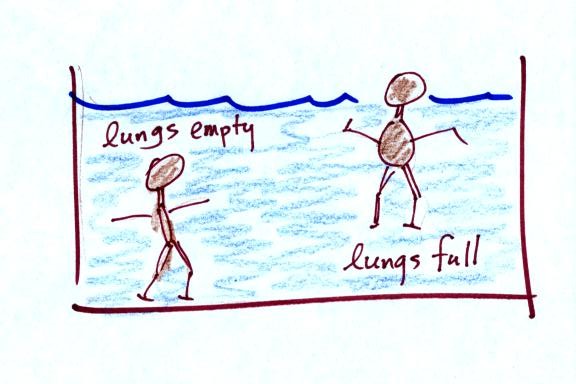
Many people can fill their lungs with air and make
themselves float, or
they can empty their lungs and make themselves sink.
People must have a density that is about the same as water.
In at least one way, people are like cans of Pepsi and Diet Pepsi.
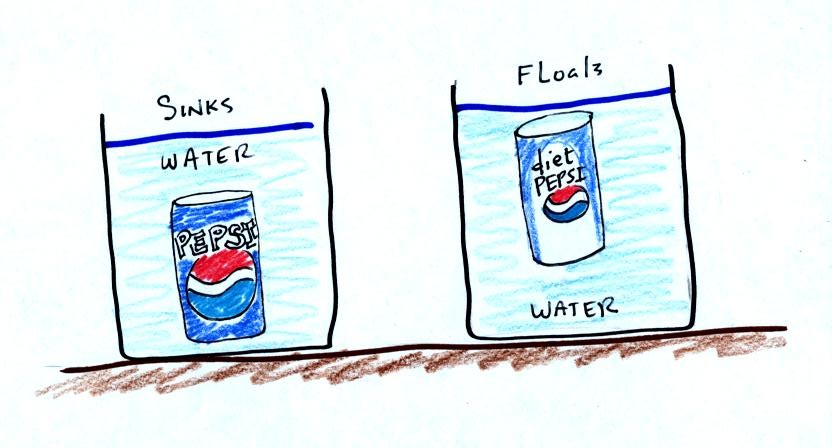
A can of regular Pepsi was placed in a beaker of
water. The can
sank.
Both cans are made of aluminum which has a density almost three times
higher than water. The drink itself is largely water. The
regular Pepsi also has a lot of sugar or corn syrup, the diet Pepsi
doesn't. The water+corn syrup mixture has a density greater than
plain
water. Both cans contain a little air (or perhaps carbon dioxide
gas). This is much less dense than water.
The average density of the can of regular Pepsi (water&sugar +
aluminum + air) ends up being slightly greater than the density of
water. The average density of the can of diet Pepsi (water +
aluminum + air) is slightly less than the density of water.
Now one
last topic, the effects of clouds on nighttime low and daytime high
temperatures. You'll find this discussed on pps 72a and 72b in
the Classnotes. The discussion below is slightly different from
the one in class.
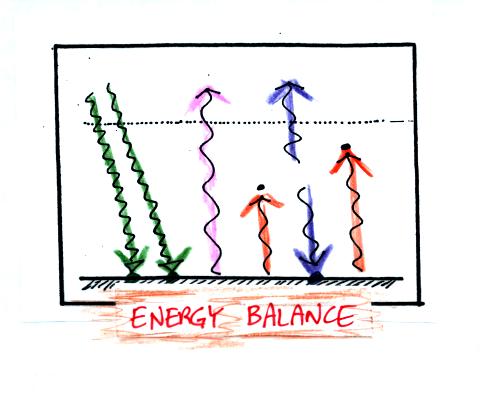
Here's the simplified picture of radiative equilibrium (something
you're probably getting pretty tired of seeing)
The two pictures below show what happens at night when you remove the
two green rays of incoming sunlight.

The picture on the left shows a clear night. The ground is losing
3 arrows of energy and getting one back from the atmosphere.
That's a net loss of 2 arrows. The ground cools rapidly and gets
cold during the night.
A cloudy night is shown at right. Notice the effect of the
clouds. Clouds are good absorbers of IR radiation. If we
could see IR light, clouds would appear black, very from what we are
used to. Now none of the IR radiation emitted by the ground
passes through the atmosphere into space. It is all absorbed
either by greenhouse gases or by the clouds. Because the clouds
and atmosphere are now absorbing 3 units of radiation they must emit 3
units: 1 goes upward into space, the other 2 downward to the
ground. There is now a net loss at the ground of only 1
arrow.
The ground won't cool as quickly and won't get as cold on a cloudy
night as it does on a clear night.
The next two figures compare clear and cloudy days.
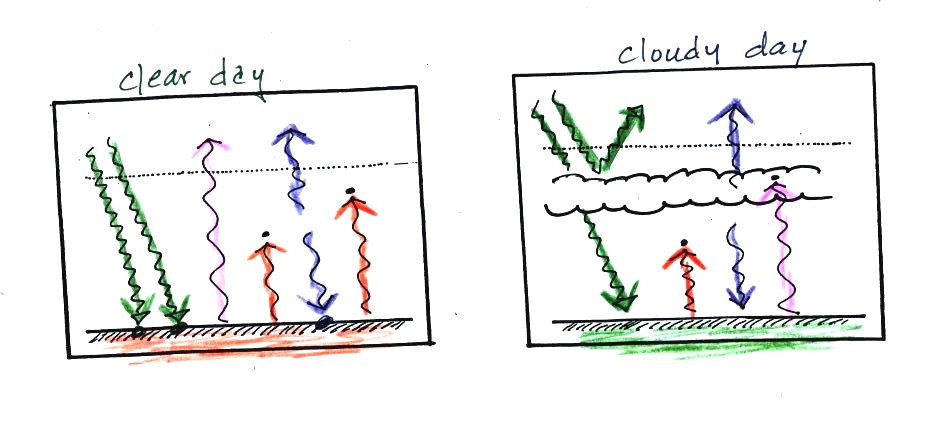
Clouds are good reflectors of visible light. The effect of this
is to reduce the amount of sunlight energy reaching the ground in the
right picture. With less sunlight being absorbed at the ground,
the ground doesn't need to get as warm to be in energy balance.
It is generally cooler during the day on a cloudy day than on a clear
day.
Clouds raise the nighttime minimum temperature and lower the daytime
maximum temperature.
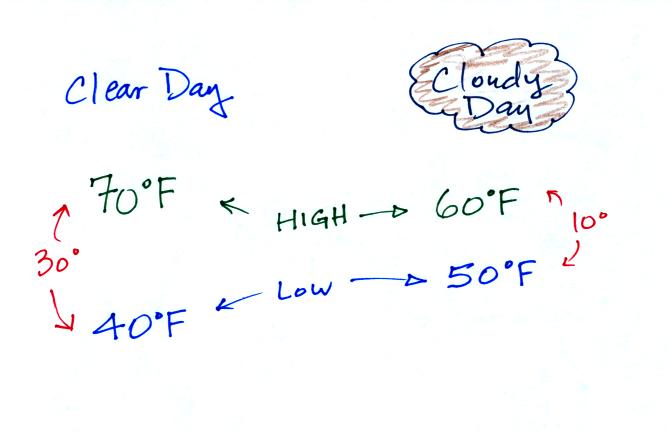
Typical daytime highs and nighttime lows in Tucson for this
time of year. Note how the clouds reduce the daily range of
temperature.
Thanks for taking some time to
read through the online notes - Have a nice remainder of the weekend