Thursday, Jan. 26, 2006
The Practice Quiz Study Guide (online
version) is now available.
The first of this semester's Optional
homework Assignments was distributed in class. The assignment
is due next Thursday, Feb. 2.
Don't forget, 1S1P Assignment #1 reports
are due next Tuesday.
Mass,
gravity, and weight are about all you need to know to start to
understand atmospheric pressure.
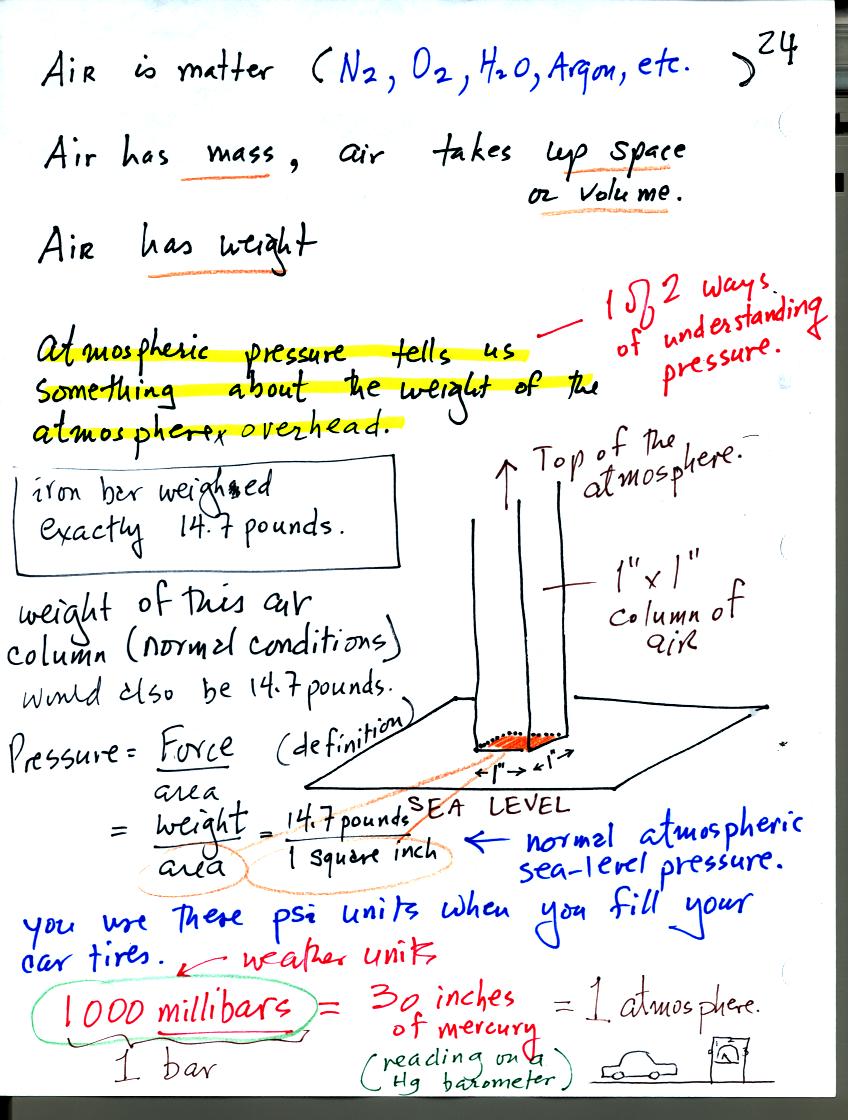
Gravity pulls downward on the air surrounding the earth producing
weight (people didn't really realize that air had mass and weight and
didn't devise ways of measuring air pressure until the
1600s).
Air pressure is determined by and tells you something
about the weight of the air overhead. This is one way of trying
to understand atmospheric pressure. It is an important concept
and will come up a lot in this course. It is worth spending
sometime to understand and remember it.
A one inch by one inch column of air extending from sea level to the
top of the atmosphere would, under average conditions, weigh 14.7
pounds (the same as the 4 foot long iron bar that was passed around in
class on Tuesday). Pressure is defined as force divided by area,
in this
case weight divided by area. So a typical sea level pressure
would be 14.7 pounds per square inch or psi. These are the same
units used when filling an automobile tire with air. You usually
put around 30 psi into car tires.
We will use millibar (mb) units in our course. Standard
atmospheric
pressure is about 1000 mb or 30 inches of mercury. The second
value refers to the reading from a mercury barometer. 1000
millibars is equal to 1 bar or 1 atmosphere.
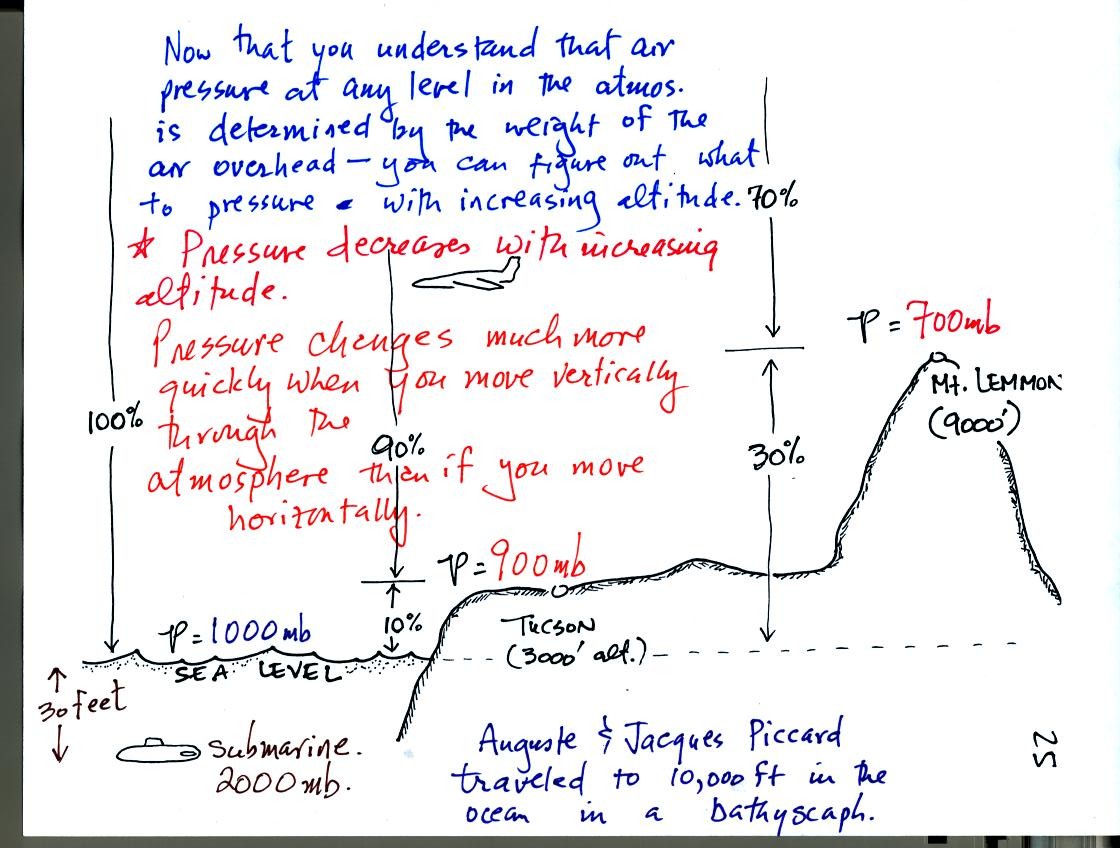
As you move upward through the atmosphere there is less and less
air
left overhead. Since the pressure at any level in the atmosphere
is
determined by the weight of the air remaining overhead, pressure
decreases with increasing altitude. Pressure changes much more
quickly when you move in a vertical direction than it does when you
move horizontally. This will be important when we cover surface
weather maps. Meterologists attempt to map out small horizontal
changes or differences in pressure on weather maps. These small
changes are what cause the wind to blow and produce weather.
Pressure increases rapidly as you descend into the ocean. The
pressure at some level in the ocean is determined by the atmospheric
pressure plus the pressure produced by the weight of the water above
you. Water is much heavier than air; pressure already be 2000
mb when you are only about 30 feet deep in the ocean.
We took a
short detour at this point and watched some more of the
video tape
about Auguste Piccard. In this segment Auguste and his son
Jacques descended to 10,000 feet depth in the ocean in a
bathyscaph. At that depth the pressure is 5000 psi.
Jacques would later descend to 35,000 feet with another
person.
That is as deep as you can go in the ocean.
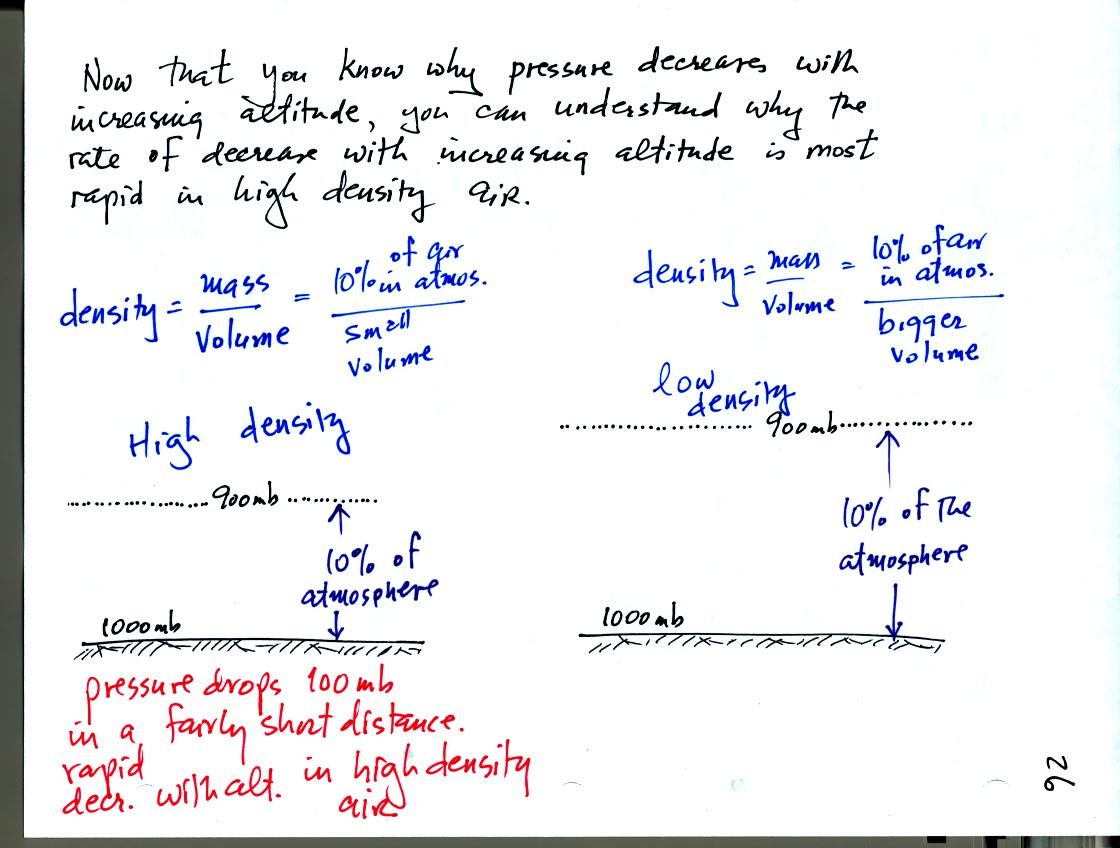
As you move upward from the ground pressure decreases by 100 mb in both
figures. Both layers contain the same amount of air (10% of the
air in the atmosphere). That air is found in a smaller volume in
the figure at left (the layer is thinner). This means the air at
left is denser than the air at right. The drop in air pressure in
the layer at left occurs in a shorter vertical distance than in the air
layer at right.
The rate of pressure decrease
with altitude is higher in the dense air at left than in the lower
density air at right.
This is a fairly subtle but important concept. We will use it
when we try to understand the intensification of hurricanes later in
the semester.
You can use bricks and wooden blocks to to visualize and understand why
pressure decreases with increasing altitude and why the rate of
decrease depends on density. You probably don't need to read this
section if you already understand those concepts.
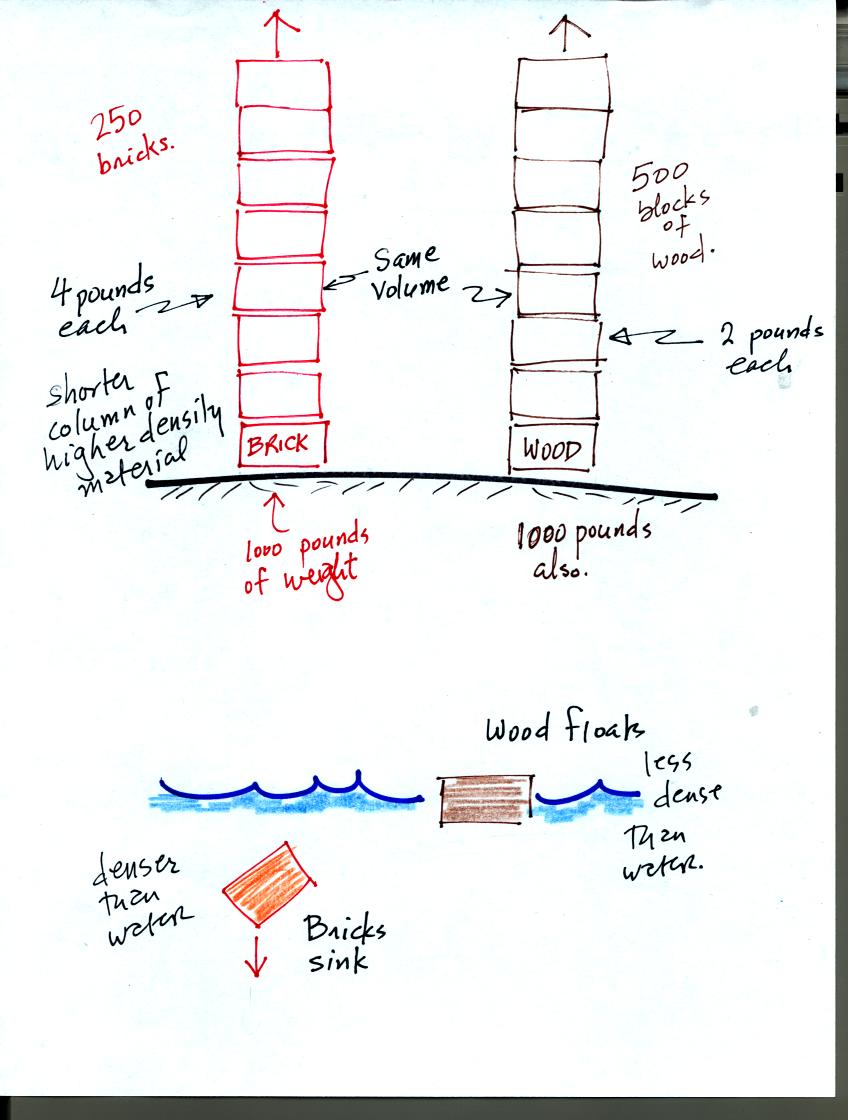
A pile of 250 bricks each weighing 4 pounds will have the same total
weight as a pile of 500 wooden blocks that weigh 2 pounds each.
The pressure at the bottoms of the two piles would be the same. A
relatively short column of high density material can produce the same
surface pressure as a taller column of lower density material.
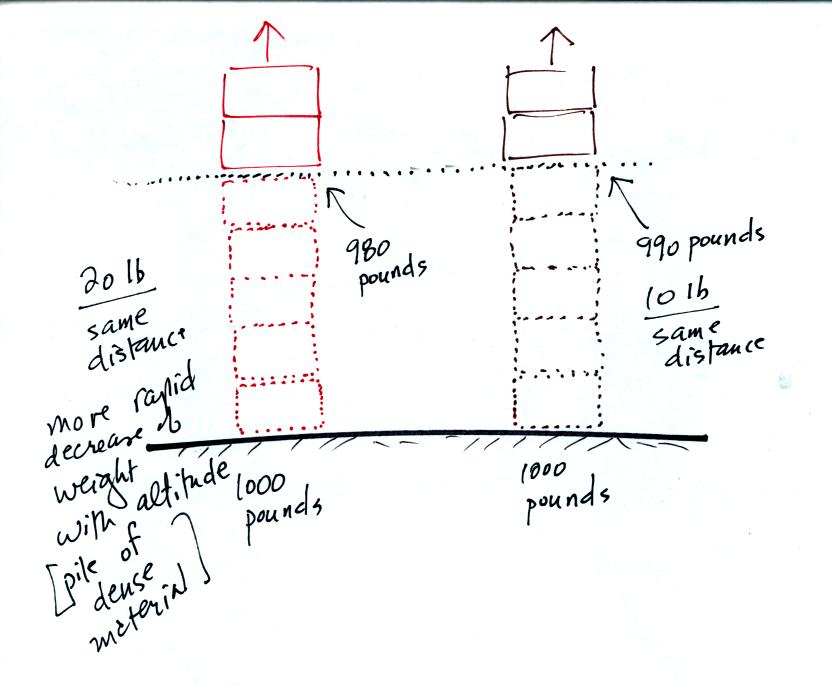
Now imagine moving upward 5 bricks in the pile at left and the same
distance, 5 blocks, in the pile at right. At left the weight will
decrease by 20 pounds, in the pile at right by 10 pounds. To find
the rate of decrease divide the decrease in weight by the distance over
which it occurred (will use the number 5 and not worry about what the
units might be).
So at left the rate of decrease is 20/5 = 4 and at right 10/5 =
2. Weight (or pressure since it is determined by weight) is
decreasing more rapidly in the high density pile of bricks than in the
lower density pile of wooden blocks.
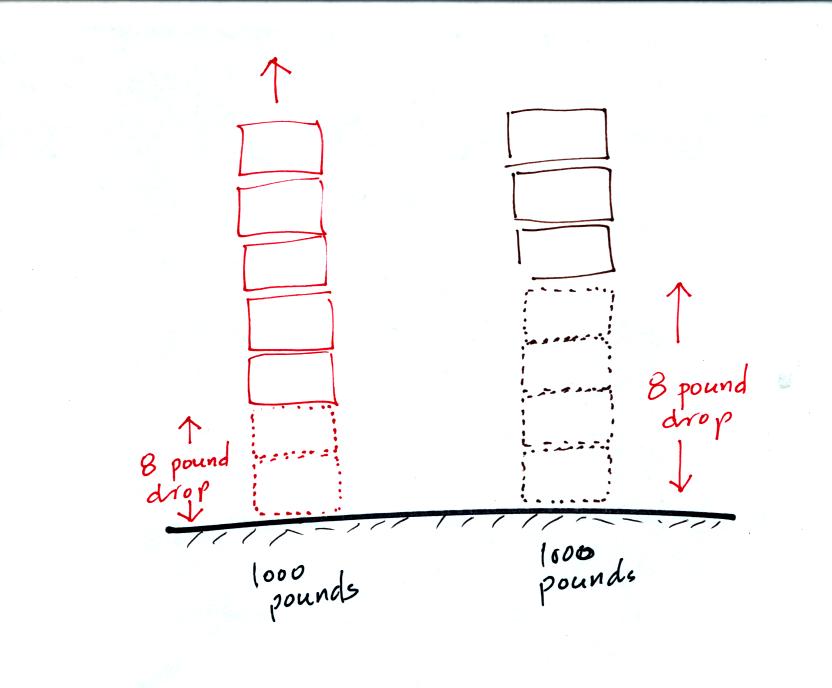
In this slightly different example you travel upward until there is an
8 pound drop in weight (up 2 bricks in the left pile, up 4 blocks in
the right pile). Again we will divide the amount of decrease by
the distance over which it occurred. For the brick pile this is
8/2 = 4. For the pile of blocks it is 8/4 = 2. We end
up with the same conclusion again, that weight (pressure) decreases
most rapidly when you move upward through high density material.
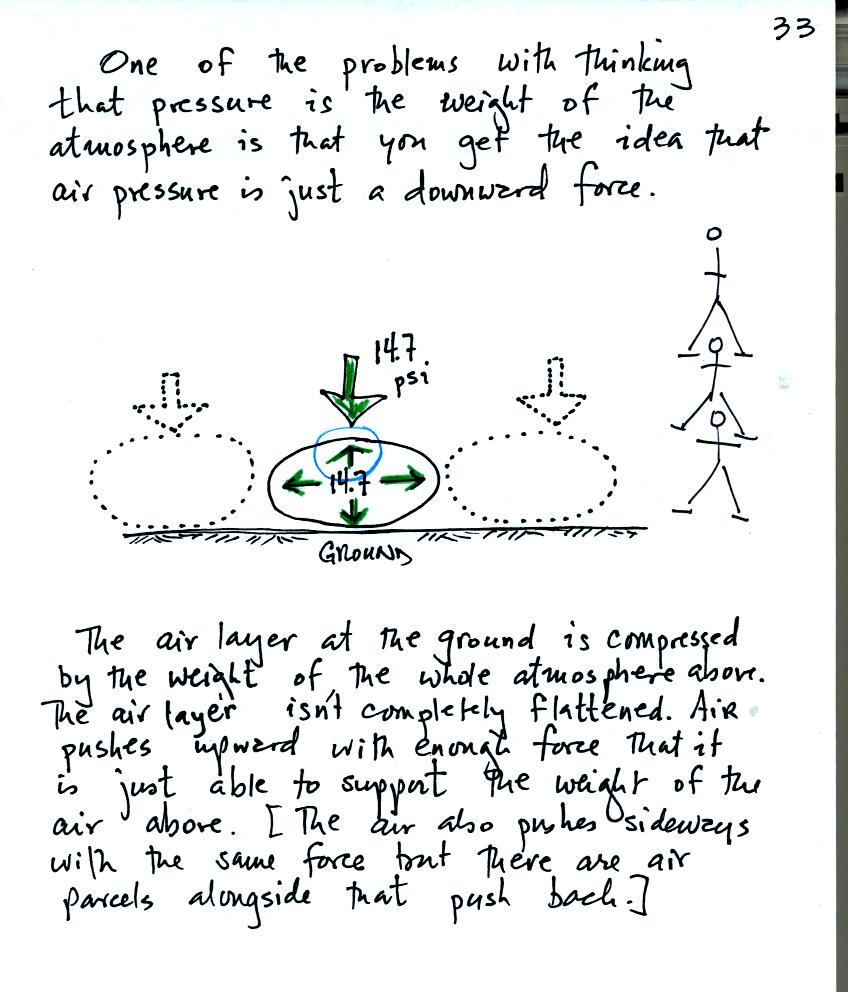
Pressure is a force that pushes downward, upward, and sideways.
The bottom person in the "people pyramid" must push upward with enough
force to support the other people. A brick at the bottom of a
pile of bricks must push upward with enough force to support the bricks
above (otherwise the bottom brick will be crushed). The air
pressure in the four tires on your automobile push down on the road
(you would feel that if the car ran over your foot) and push upward
with enough force to keep the 1000 or 2000 pound vehicle off the
road.
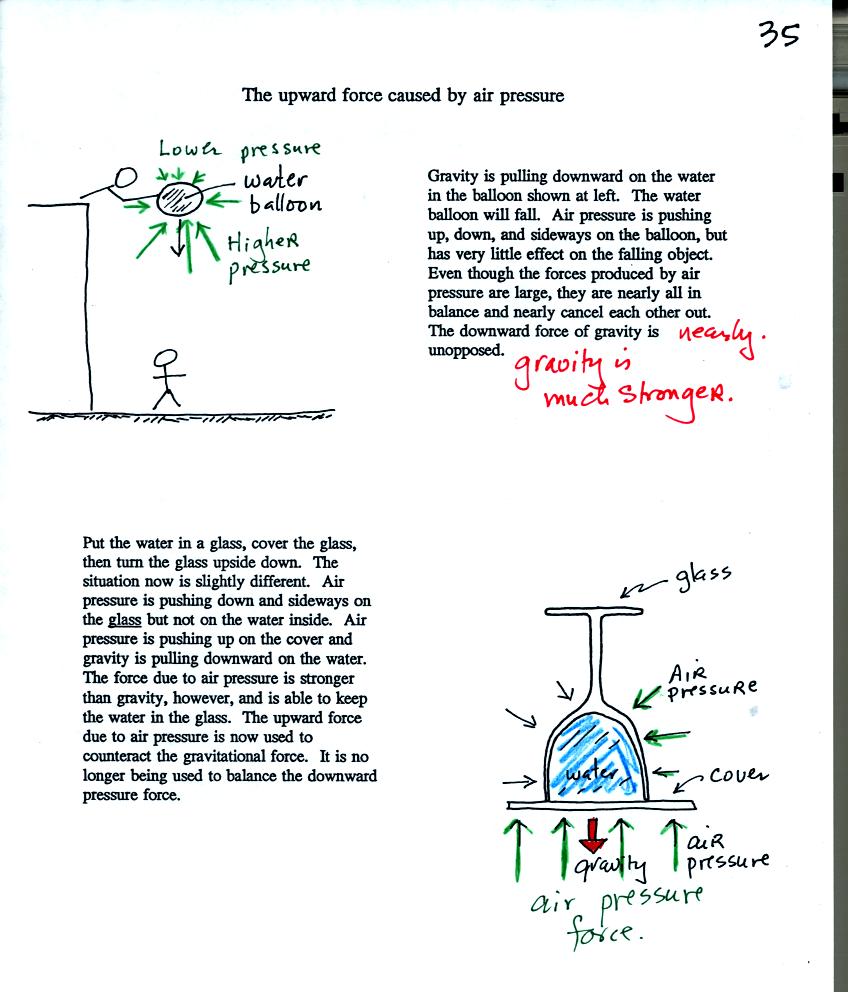
I am going to redraw the two figures from p. 35 in the photocopied
notes that described the class demonstration of upward air pressure
force. Hopefully this will make for a clearer explanation of the
demonstration.
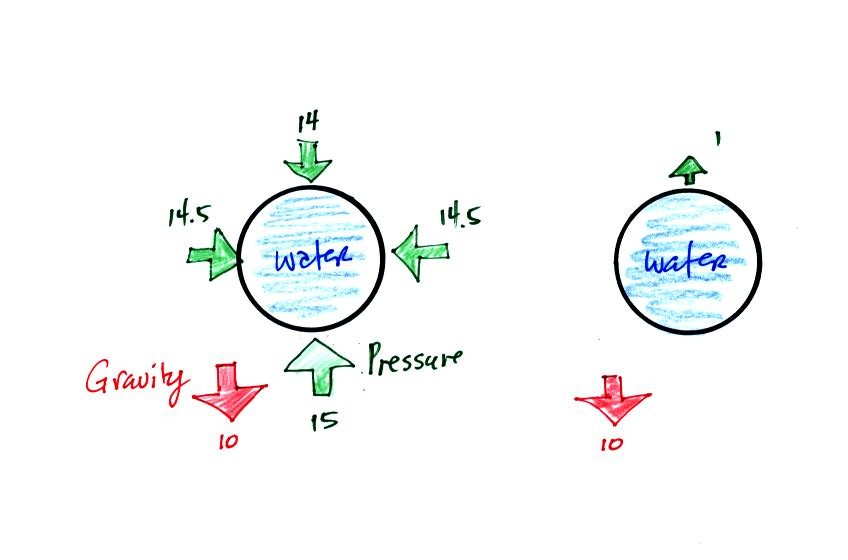
At left, gravity, the red arrow, pulls downward on the water
balloon. The 10 is the strength of the gravitational force (I
just made this number up, don't worry about the units either).
Air pressure pushes upward on the bottom of the balloon with strength
15, slighly weaker forces (14.5) push sideways, and at the top of
the balloon air pressure pushes downward with strength 14.
Pressure decreases with increasing altitude, that is why the pressure
forces get weaker as you move from the bottom to the top of the
balloon.
Notice the two sideways forces cancel each other out. The upward
pointing pressure force is slightly stronger than the downward pressure
force. They don't quite cancel each other out, you are left (as
shown in the figure at right) with an upward pointing pressure
difference force of strength 1. Gravity (10) is stronger than the
pressure difference force (1) and the water balloon falls.
Now we'll look at the situation for the water in an upside down wine
glass
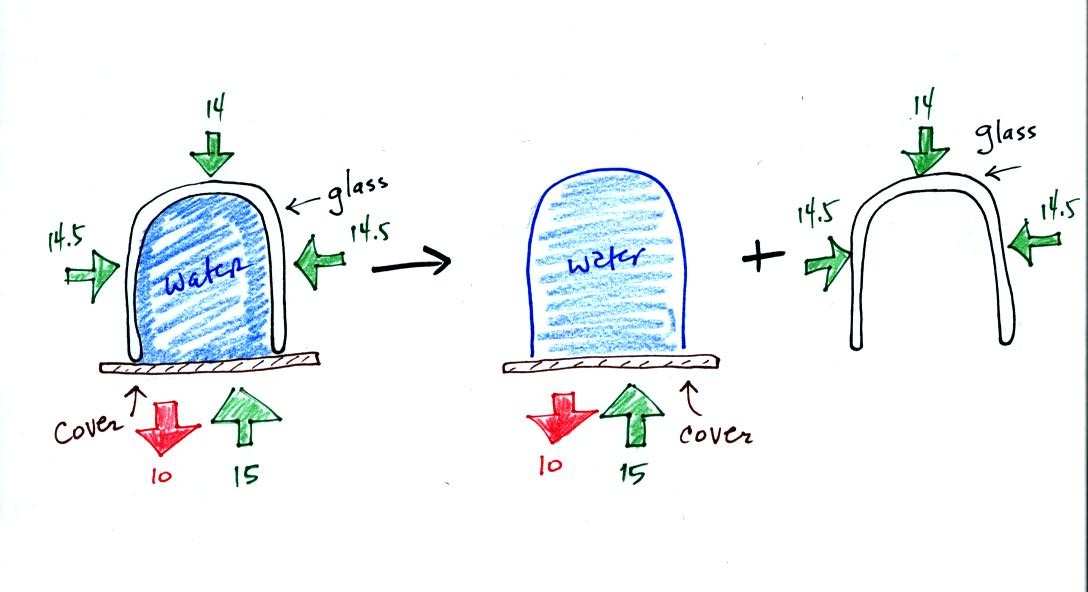
The figure at left shows the wine glass (the stem of the glass has been
left off the drawing), the plastic cover, and the pressure and gravity
forces.
You can split this into two parts: (1) gravity pulling downward
and the pressure force pushing upward on the cover and water in the
glass and (2) the pressure force pushing downward and sideways on the
glass (which the instructor was holding in his hand). Now
notice that the 15 unit pressure force pushing up on the water is
greater than the 10 units of gravity pulling downward. The upward
pressure force now can keep the water from falling out of the glass.
Here's another situation where pressure and gravity forces are battling
each other for dominance, a balloon.
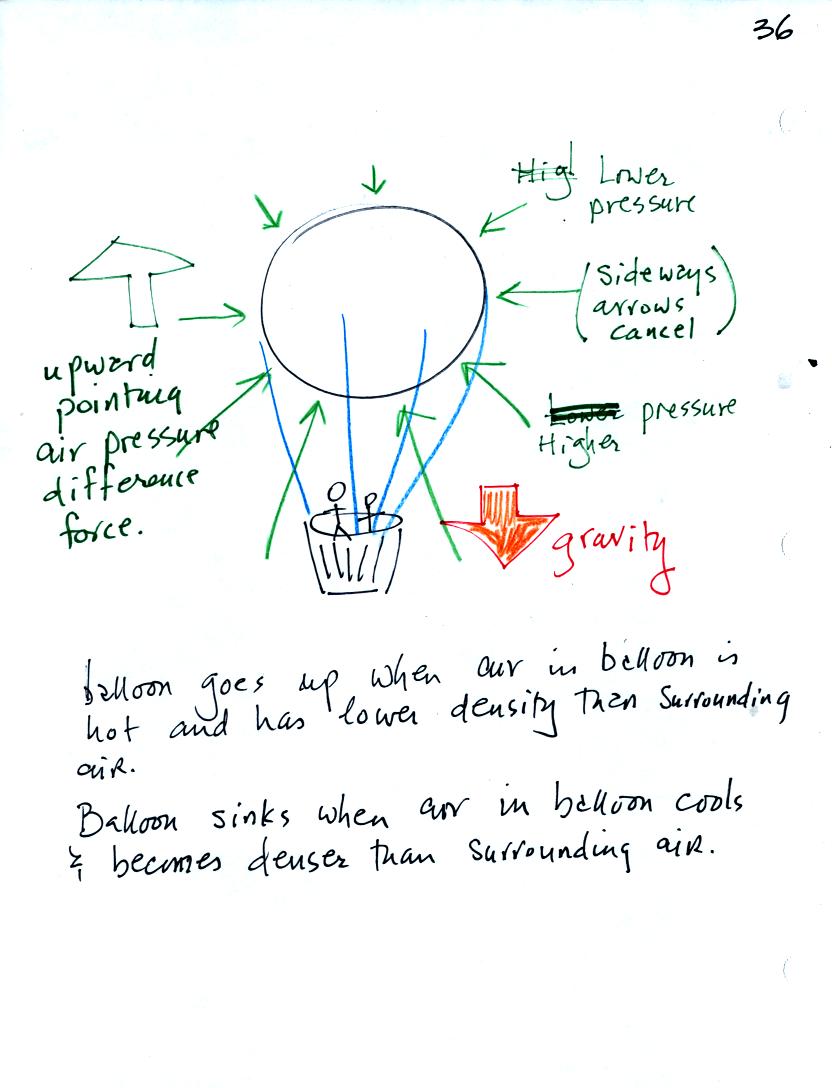
This is like a water balloon except that the balloon is now filled with
a gas like air or helium. This gas is much lighter than
water. The gravity force will be much weaker and the upward
pressure difference force (the difference between the upward point
force at the bottom of the balloon and the downward pointing force at
the top of the balloon) will sometimes end up stronger than gravity.
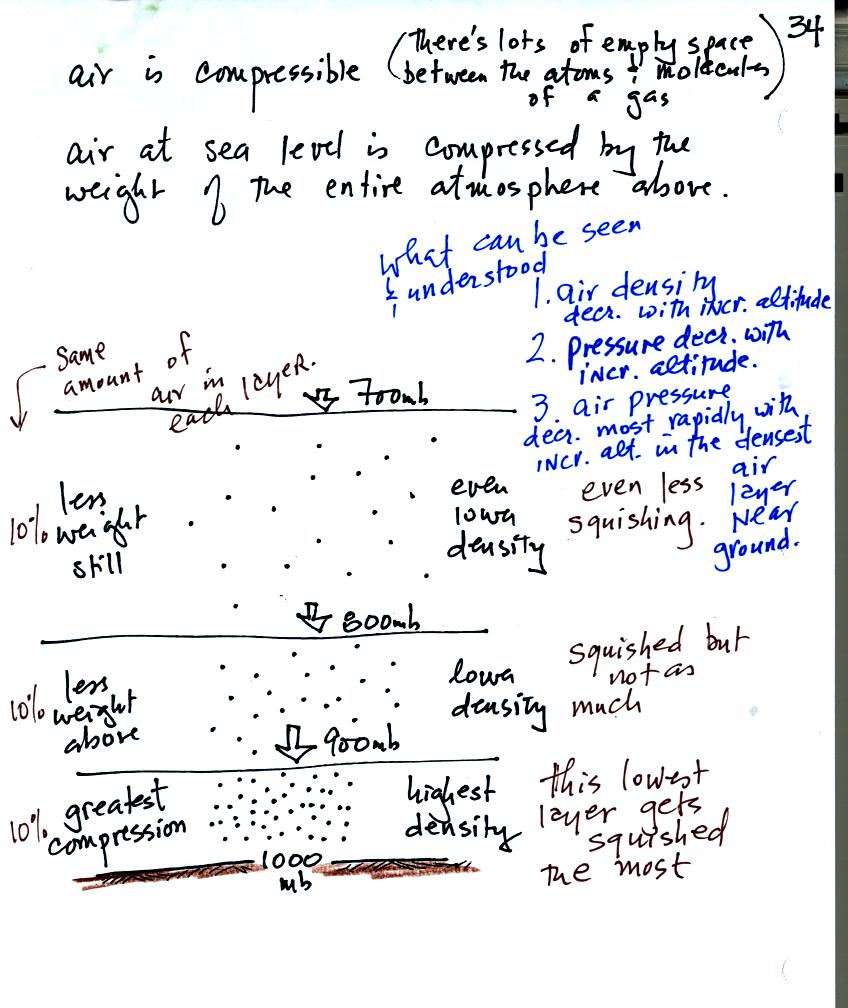
Three layers of air in the atmosphere are shown above (each layer
contains the same amount of air, 10% of the air in the
atmosphere). The layer at the ground and at the bottom of the
atmosphere is "squished" by the weight of the air above.
Squeezing all of this air into a thin layer or small volume increases
the air's density.
The next layer up is also squished but not as much as the bottom
layer. The density of the air in the second layer is lower than
in the bottom layer. The air in the 3rd layer has even lower
density. It is fairly easy to understand that air density
decreases with increasing altitude.
This figure also reminds you that air pressure decreases with
increasing altitude.
If you look carefully you see again that the most rapid rate of
pressure decrease with increasing altitude occurs in the thin layer of
high density air next to the ground.