Thursday Oct. 3, 2019
Ravel's "Bolero"
played by the Copenhagen Philharmonic Orchestra (Sjællands
Symfoniorkester) at Copenhagen's Central Railway Station
(4:52), Gustav Holst "The Planets:
Jupiter The Bringer of Jollity" Berklee Contemporary
Symphony Orchestra at The Prudential Center (4:22), Edvard Grieg's
"In the Hall
of the Mountain King" Centre Commercial Auchan Noyelles,
Lille, France (6:00), Henri Herbert "Henri's
Boogie" (6:36)
You'll need page
45a, page
45b, page
46a, page 47a, page 47b, page 48a, and page 48b. I'm going
to print out page 55, page 56, page 57, and page 58 also just in case we
had some extra time to get into that material (in the end we did
not).
There's a lot of material below.
Fortunately we were able to do a little of it, Points 1 - 3, at
the end of class on Tuesday. We'll jump straight to Point 4.
It's important to keep all of this organized. So I've
numbered the various topics and have put together a summary of the
main points. You'll find the summary at the end of today's
notes
1. Types of energy (see page
43 in the ClassNotes)
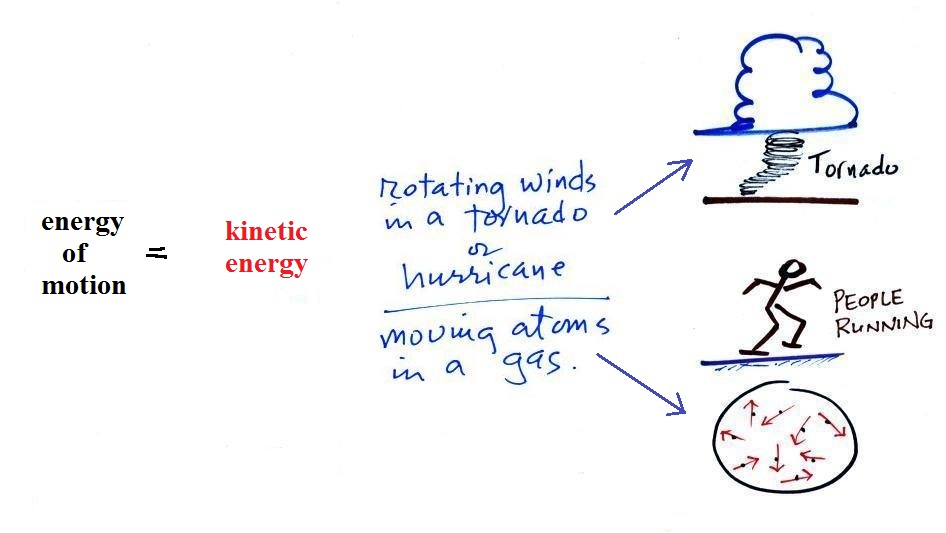
Kinetic
energy is energy of motion.
Some examples (both large and microscopic scale) are
mentioned and sketched above. This is a relatively
easy to visualize and understand form of energy.
Radiant energy This is probably the
most important form of energy that we'll be dealing with.
Electromagnetic radiation is another name for radiant
energy. Sunlight is an example of radiant energy.
Radiant energy is something that we can see and feel (you feel
warm when you stand in sunlight). Something that is not
quite so obvious is that everyone in the classroom is emitting
radiant energy. This is infrared light, an invisible form
of radiant energy. And actually it's not just the people;
the walls, ceiling, floor and even the air in the classroom are
also emitting infrared light. We can't see it and, because
it's there all the time, I'm not sure whether we can feel it or
not.
Latent heat energy is
an important, under-appreciated, and rather
confusing type of energy. The word latent
refers to energy that is hidden. That's part
of the problem. Another part of
what makes latent heat energy hard to visualize and
appreciate is that the energy is hidden or stored in
water vapor or water - that seems like an unlikely place
to find energy.
2. We'll get the topic of energy
units out of the way
Joules
are the units of energy that you would probably
encounter in a physics class. Your
electric bill shows the amount of energy that
you have used in a month's time, the units are
kilowatt-hours.
We'll usually be using calories as units of
energy. 1 calorie is the energy need to warm 1
gram of water 1 C (there are about 5 grams of water in a
teaspoon).
3.
Temperature provides a measure of the average
kinetic energy of the atoms or molecules in a
material. Much of what follows is on page
44 in the ClassNotes)
The atoms or
molecules inside the warmer object will be moving more
rapidly (they'll be moving freely in a gas, just
"jiggling" around while still bonded to each other in
a solid). Since kinetic energy is energy
of motion, temperature gives you an idea of the
average speed of the moving atoms or molecules in a
material.
You need to be careful what temperature scale
you use when using temperature as a measure of average
kinetic energy. You must use the Kelvin
temperature scale because it does not go below zero (0
K is known as absolute zero). The smallest amount of
kinetic energy you can have is zero kinetic
energy. There is no such thing as negative
kinetic energy.
There
are three temperature scales that we might have
occasion to use in this class. They're
shown below. There are two temperatures that
you should try to remember on each scale.
The boiling and freezing points of water
on both the Celsius and the Fahrenheit scales (the freezing
point of water and the melting point of ice are the
same). Remember that the Kelvin scale doesn't go below
zero. 0 K is referred to as absolute zero, it's as cold
as you can get. A nice round number of the average
temperature of the earth is 300 K, that's the last temperature
value to remember.
Here's some additional temperature data that I'm including
just in case you're interested.
You certainly don't need to try to
remember all these numbers. The world high temperature
record value of 136 F above was measured in Libya at a
location that was only about 35 miles from the Mediterranean
coast. Water, as we will see, moderates climate, it
reduces the extremes, so it seems odd that such a high
temperature would have been recorded there. The World
Meteorological Organization recently decided the 136 F
reading was invalid and the new world record is the 134 F
measurement made in Death Valley. There is also some
question about the 134 F Death Valley value (see this
article in Wikipedia). There seems to be some
agreement that 129 F is the highest reliable measurement of
temperature. Temperatures that hot have been measured
at multiple locations.
The continental US cold temperature record of -70 F was
set in Montana and the -80 F value in Alaska. The
world record -129 F was measured at Vostok station in
Antarctica. This unusually cold reading was the result
of three factors: high latitude, high altitude, and location
in the middle of land rather than being near or surrounded
by ocean (again water moderates climate, both hot and
cold).
Liquid nitrogen is very cold but it is still quite a bit
warmer than absolute zero. Liquid helium gets within a
few degrees of absolute zero, but it's expensive and there's
only a limited amount of helium available. So I would
feel guilty bringing some to class; plus I don't think it
would look any different than liquid nitrogen.
We started class
today at this point
4. Energy, temperature, and specific heat
When you add energy to an object, the object will usually
warm up (or if you take energy from an object the object will
cool). It is relatively easy to come up with an equation
that allows you to figure out what the temperature change will
be (this is another equation I'll try to remember to write on
the board before the next quiz. Try to understand
it, you don't have to memorize it.
The temperature change, ΔT, will first depend on how
much energy was added, ΔE. This is
a direct proportionality, so ΔE is in the
numerator of the equation (ΔE and ΔT
are both positive when energy is added, negative when energy is
removed)
When you add equal amounts of energy to large and small pans
of water, the water in the small pan will get hotter. The
temperature change, ΔT, will depend on
the amount of water, the mass. A small mass will mean a
large ΔT, so mass should go in the
denominator of the equation.
Specific heat is what we use to account for the fact that
different materials react differently when energy is added to
them. A material with a large specific heat will warm more
slowly than a material with a small specific heat.
Specific heat has the same kind of effect on ΔT
as mass. Specific heat is sometimes called "thermal mass"
or "thermal capacity."
Here's an important example that will show the effect of
specific heat (see page
45b in the ClassNotes).
Equal amounts of energy (500 calories) are added
to equal masses (100 grams) of water and soil. We use
water and soil in the example because most of the earth's
surface is either ocean or land. Before we do the calculation,
try to guess which material will warm up the most.
Everything is the same except for the specific heats. Will
water, with its 4 times larger specific heat, warm up more or
less than the soil?
The details of the calculation are shown below.
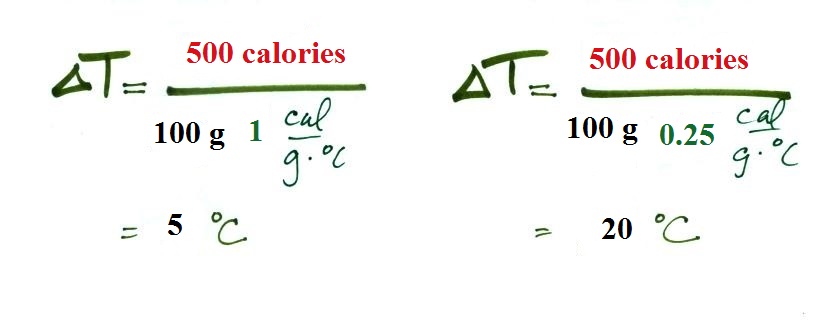
With its higher specific heat, the water doesn't heat up
nearly as much as the soil. If we had been removing
energy the water wouldn't cool off as much as the soil
would.
5. Water moderates climate
These different rates of warming of water and soil have
important effects on regional climate.
Oceans moderate the climate. Cities near a large body
of water won't warm as much in the summer and won't cool as much
during the winter compared to a city that is surrounded by land.
Water's ΔT is smaller than land's because water
has a higher specific heat.
The yearly high and low monthly average temperatures are
shown at two locations above. The city on the coast has a
30o F annual range of temperature (range is
the difference between the summer and winter
temperatures). The city further inland (assumed to be at
the same latitude and altitude) has an annual range of
60o F. Note that both cities have the same 60o
F annual average temperature.
Water moderates climates - it reduces the difference between
summertime high and wintertime low temperatures.
Growing tomatoes in the desert -
practical application
Here's another situation where you can take
advantage of water's high specific heat to moderate climate on a
smaller scale (it fits better in the Spring semester edition of
the class than the Fall semester).
This is my winter vegetable
garden (brocolli plants can be seen in the background).
Tomatoes (foreground) are a summer vegetable. in Tucson
you need to get tomatoes started early so that they can
produce fruit before it gets too hot. I usually start
mine in February and you need to protect the plants from
frost.
Here's one way of doing that. You
moderate the climate and surround each plant with a "wall o
water" - a teepee like arrangement that surrounds
each plant. The cylinders are filled with water and they
take advantage of the high specific heat of water and won't cool
as much as the air or soil would during a cold night. The
walls of water produce a warm moist micro climate that the
tomato seedlings love. The plastic is transparent so
plenty of sunlight can get through. Note the brocolli
growing in the background, it isn't nearly as sensitive to the
cold and doesn't require protection.
6. Energy
transport processes
By far the most important process is at the bottom of the list
above. Energy transport in the form of electromagnetic
radiation (sunlight for example) is the only process that can
carry energy through empty space. Electromagnetic radiation
travels both to the earth (from the sun) and away from the earth
back into space. Electromagnetic radiation is also
responsible for about 80% of the energy transported
between the ground and atmosphere.
You might be surprised to learn that latent heat is the second
most important transport process. The term latent heat can
refer to both a type of energy and an energy transport process
(the energy is hidden in the water vapor, the water vapor can move
around and carry that energy with it).
Rising parcels of warm air and sinking parcels of cold air are
examples of free convection. Because of convection you feel
colder or a cold windy day than on a cold calm day (the wind chill
effect). Ocean currents are also an example of
convection.
Convection is also one of the ways of rising air motions in the
atmosphere (convergence into centers of low pressure and fronts
are two other ways we've encountered so far). Caution:
convection is 1 of 4 energy transport processes and 1 of 4
processes that cause rising air motions.
Conduction is the least important energy transport at least in
the atmosphere. Air is such a poor conductor of energy that
it makes a very good insulator.
7. Energy
transport by conduction
Conduction is the first of four energy transport processes that we
will cover (and the least important transport process in the
atmosphere). The figure below illustrates this
process. Imagine heating the end of a piece of something
just so you can visualize a hot object. If you held the
object in air it would slowly lose energy by conduction and cool
off.
How does that happen? In the top
picture some of the atoms or molecules near the hot object
are colliding with the object are picking up energy.
In the middle picture the initial layer of molecules are
colored orange. They are moving faster than they
were. They begin to collide with their outer neighbors
and start to share energy with them.
In the third picture molecules further out (yellow) have
now gained some energy. The random motions and
collisions between molecules is carrying energy from the hot
object out into the colder surrounding air.
Conduction transports energy from hot to cold. The
rate of energy transport depends first on the temperature
gradient or temperature difference between the hot object
and the cooler surroundings. If the object in the
picture had been warm rather than hot, less energy would
flow and energy would flow at a slower into the surrounding
air. If there were no temperature difference there
wouldn't be any energy transport at all.
Conduction is some ways is like diffusion. I sprinkled
a little curry powder on the table in the front of the
classroom. I hoped that random air motions could carry
(diffuse) the curry smell to the back of the room.
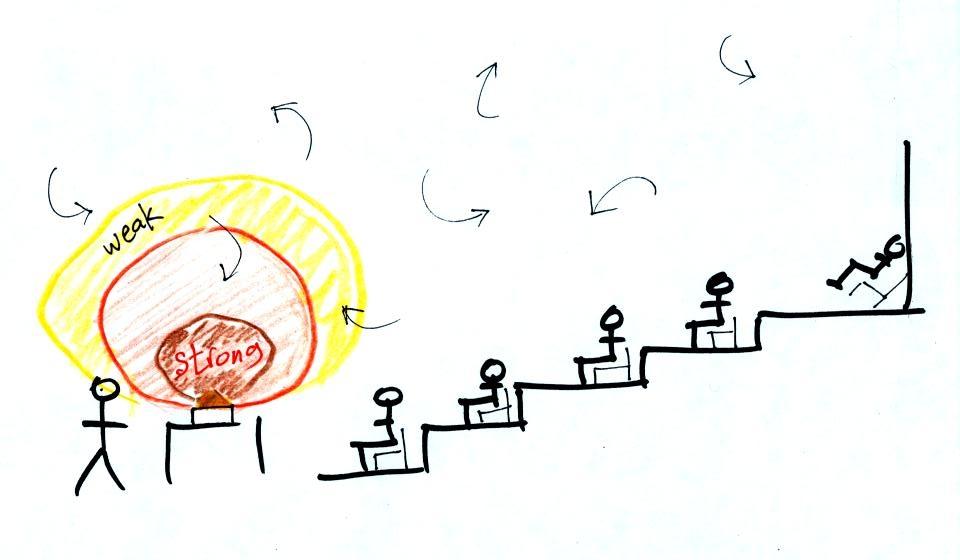
|
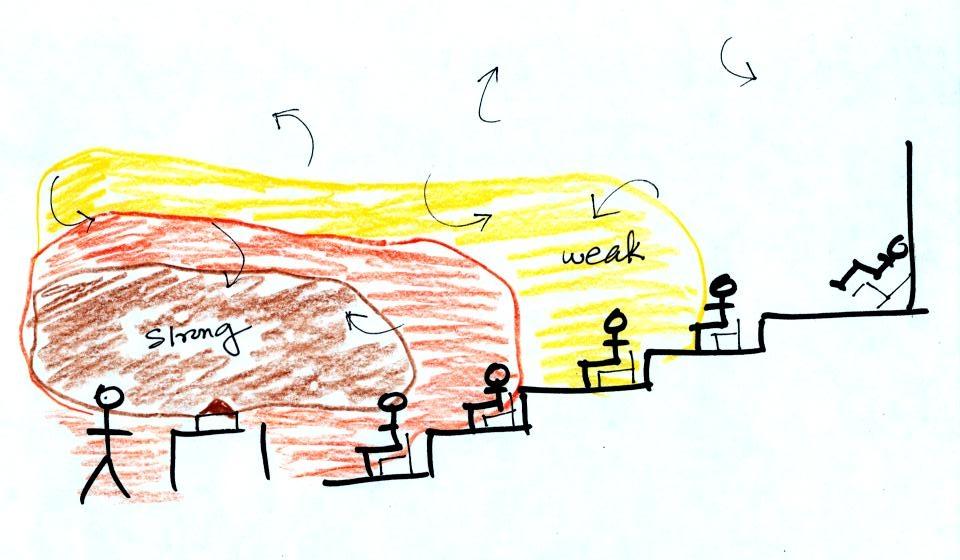
|
I hoped the smell of curry was strong
enough that air currents in the room would carry the
smell to the back of the room.
|
The smell really only made it to the
first or second row. ILC 150 is too big and the
ventilation system is too good.
|
The demonstration doesn't
really work. That's OK though because I'll add a 2nd
element to the demonstration that I hope will help you
understand the difference between conduction and convection.
The rate of energy transport by conduction also depends
on the material transporting energy (air in the example
above). Thermal conductivities of some common
materials are listed. Air is a very poor conductor of
energy and is generally regarded as an insulator.
Water is a little bit better conductor. Metals are
generally very good conductors (cooking pans are often made
of stainless steel but have aluminum or copper bottoms to
evenly spread out heat when placed on a stove).
Diamond has a very high thermal conductivity (apparently the
highest of all known solids). Diamonds are sometimes
called "ice." They feel cold when you touch
them. The cold feeling is due to the fact that they
conduct energy very quickly away from your warm fingers when
you touch them.
I brought a propane torch (2 of them actually, one to serve
as a backup) to class to demonstrate the behavior of materials
with different thermal conductivities. Here's
what I wanted to illustrate
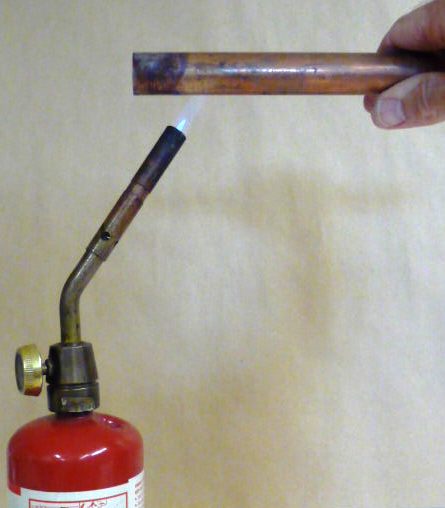
|
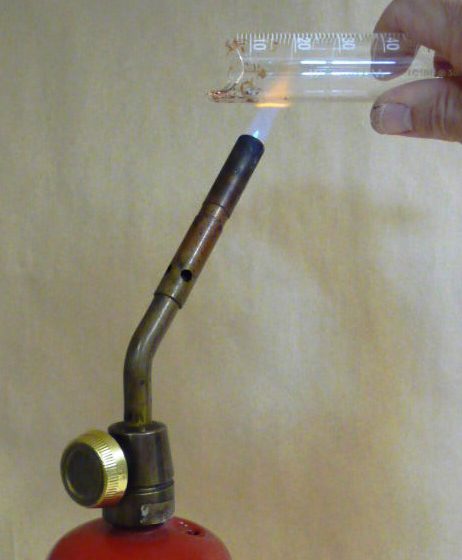
|
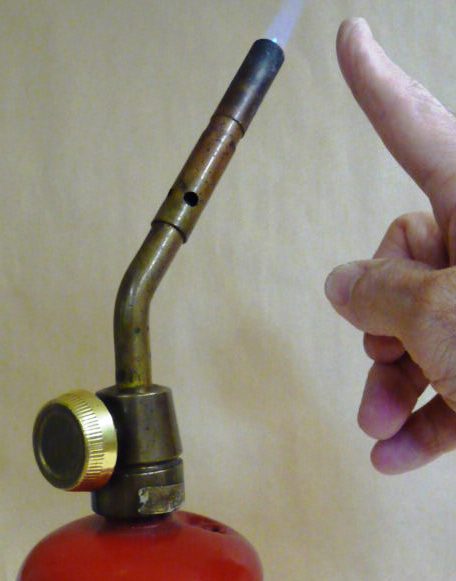
|
Copper is a good
conductor. You must move your fingers several
inches away from the end to keep from getting burned.
|
Glass has much lower thermal
conductivity. You can hold onto the glass just a
couple of inches from the flame and not feel any
heat. Because energy is not being carried away
from the end of the piece of glass, the glass can get
hot enough to begin to glow red.
|
You can put your finger
alongside the flame with just 1/2 inch or so of
separation. Air is a very poor conductor.
Don't put your finger above the flame though.
|
Because air has such a low thermal conductivity it is often
used as an insulator.
It is important, however, to keep the air trapped in small pockets
or small volumes so that it isn't able to move and transport
energy by convection (we'll look at convection momentarily).
Here are some examples of insulators that use air:
Foam is often used as an
insulator. Foam is filled with lots of small
air bubbles, that's what provides the insulation.
You
can safely hold onto a foam cup filled with liquid nitrogen (-320 F)
because the foam does such a good job insulating
your fingers from the cold liquid inside.
Thin insulating layer of air in a double pane
window. I
don't have double pane windows in my house.
As a matter of fact I leave a window open so my
cats can get in and out of the house (that's not
particularly energy efficient). It also
means there are lots of mosquitoes in the house in
the summer.
We really
haven't needed winter coats yet in Tucson this
semester.
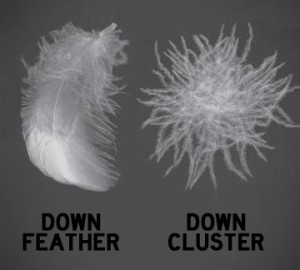
|
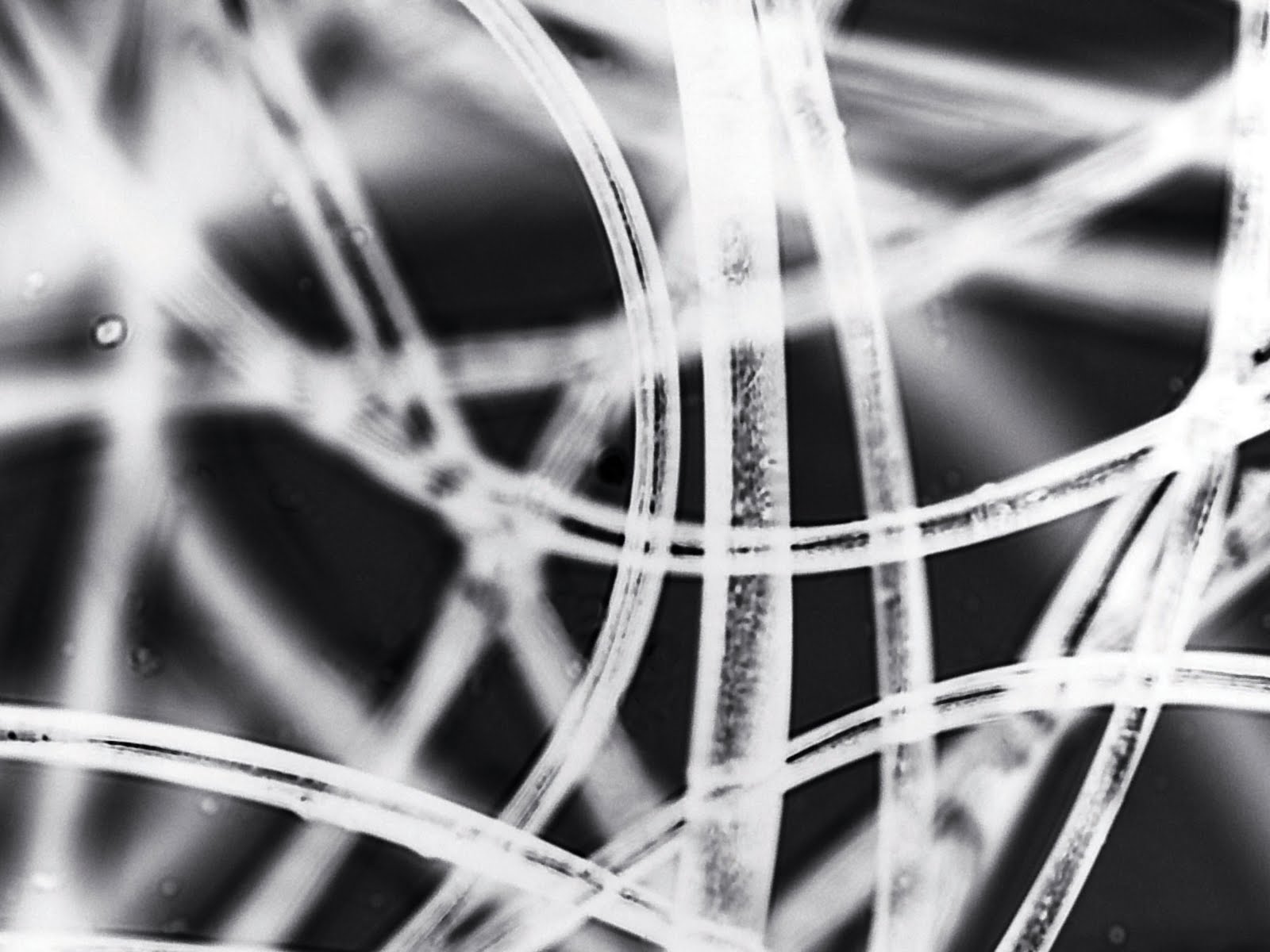
|
Down feathers are
often used in coats and sleeping bags.
Packing together a bunch of the "clusters"
produces very good insulation provided the
feathers stay "fluffed up" and trap
air. source
of this image |
Synthetic fibers (Primaloft -
Synergy are shown above in a microphotograph) have
some advantages over down. There is still
some insulation when wet and the material is
hypoallergenic. source
of this image |
|
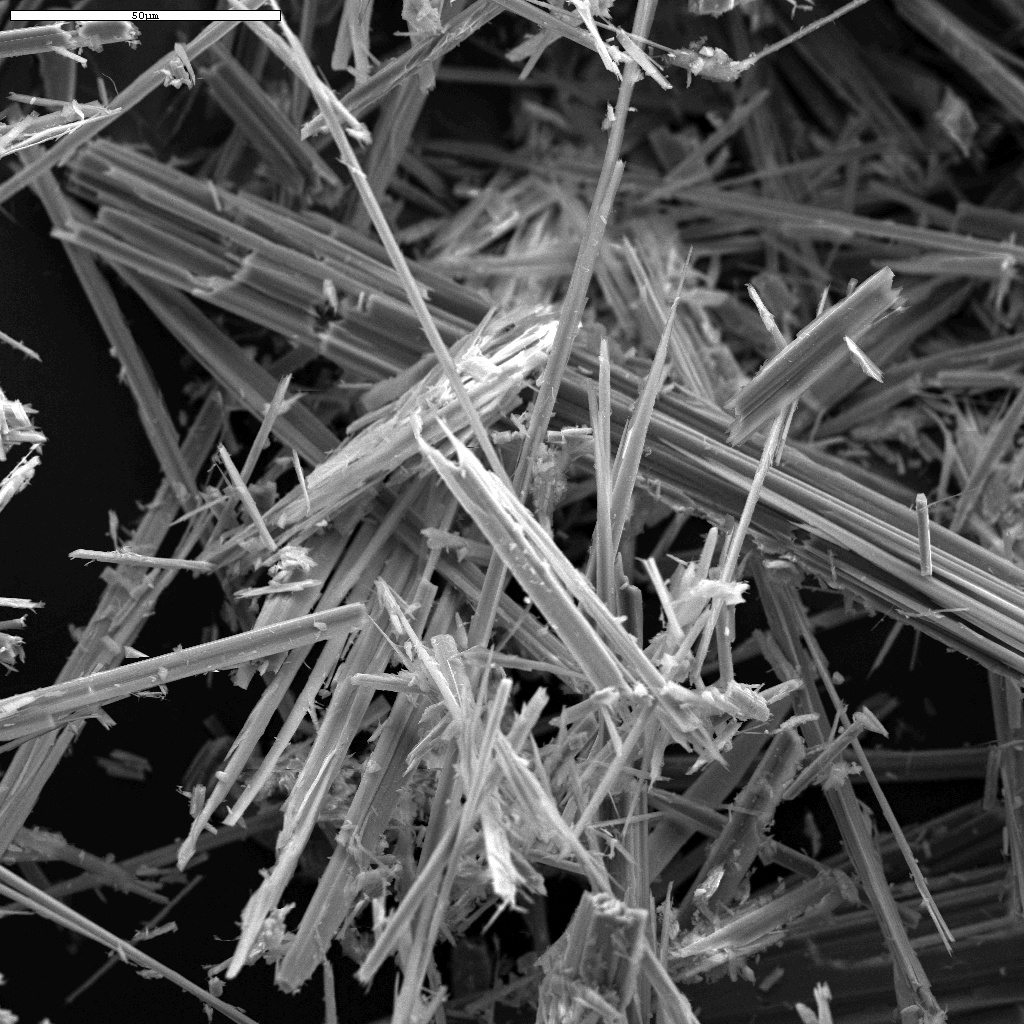
|
A
photograph of aerogel (image source),
sometimes known as solid air. It's an
excellent insulator because it is mostly
air. The very small particles in
the aerogel are scattering light in the same way air
molecules do. That's why it has this sky blue
color.
|
A scanning
electron microscope photograph of asbestos
which was once widely used as insulation.
Asbestos fibers can cause lung cancer and
other damage to your lungs when inhaled.
The white bar at the top left edge of the
image is 50 um across.
You can find this image and read
more about asbestos here.
|
8. Energy transport by
convection
I used the torch again to heat up the broken glass graduated
cylinder. The glass gets so hot that you can see it starting
to glow red.
How would you cool off a hot object like this? You could
just hold onto it and it would eventually cool by
conduction. If you were in a little bit more of a
hurry you could blow on it. That's forced convection,
the energy transport process we will be covering next. Or
you could stick the hot end of the cylinder into some water (you'd
hear a short hissing sound and the glass would probably
shatter). The hissing would mean the hot piece of glass had
evaporated some water. That would be an example of latent
heat energy transport which we'll be discussing later in the
period.
Rather than moving about randomly, the atoms or molecules move
together as a group (organized motion). Convection works in
liquids and gases but not solids (the atoms or molecules in a
solid can't move freely).
In the middle of the picture
above a thin layer of air surrounding a hot object has been
heated by conduction. Then a person is blowing the blob of warm
air off to the right. The warm air molecules are moving
away from the hot object together as a group (that's the
organized part of the motion). Cooler air moves in and
surrounds the hot object and the whole process repeats
itself.
At this point I put a small fan behind the curry
powder with the idea that the fan would carry the curry smell
further back in the room. I'm not sure how far back the
smell traveled.
And actually you don't need to force convection, it will
often happen on its own.
A thin layer of air in the figure above (lower left) is heated
by conduction. Then because hot air is also low density
air, it actually isn't necessary to blow on the hot object, the
warm air will rise by itself.. Energy is being transported
away from the hot object into the cooler surrounding air.
This is called free convection. Cooler air moves in to
take the place of the rising air and the cycle repeats itself.
The example at upper right is also free convection. Room
temperature air in contact with a cold object loses energy and
becomes cold high density air. The sinking air motions that
would be found around a cold object have the effect of
transporting energy from the room temperature surroundings to the
colder object.
In both examples of free convection, energy is being
transported from hot toward cold. See if you can
draw in some arrows in the two cases above showing the direction
of energy transport then click here.
I could put my finger alongside the flame from the propane
torch without any problem. There's very little energy
transported sideways through air by conduction.
Be careful if you put your
finger or hand above the torch. That's
because there's a lot of very hot air rising from the
torch. This is energy transport by free convection
and its something you can sometimes see.
Up at the front of the classroom you might have
been able to see (barely) the
shimmering of hot rising air when I held the torch in
front of the projector screen. There is a
technique, called Schlieren photography, that can
better catch these barely visible air motions (it is
able to see and photograph the differences in air
density). The photo at right is an example and
shows the hot rising air above a candle.
The photo was taken by Gary Settles from Penn
State University and can be found at this
site.
9. Real world examples of energy transport by
conduction and convection (see page
48a in the ClassNotes)
Now some examples of conductive and convective energy
transport. They really do show up in a lot more everyday
situations than you might expect.
Note first of all there is a temperature difference between
your hand and a room temperature (70 F) object. Energy will
flow from your warm hand to the colder object. Metals are
better conductors than wood. If you touch a piece of 70 F
metal it will feel much colder than a piece of 70 F wood, even
though they both have the same temperature. A piece of 70 F
diamond would feel even colder because it is an even better
conductor than metal. I brought a piece of aluminum and a
piece of wood (oak) to class so that you could check this out for
yourself.
Something that feels cold may not be as cold as it seems.
Our
perception of cold
is more an indication of how quickly our body
or hand is losing energy
than a reliable measurement of temperature.
Here's another
example
It's pleasant standing outside on a nice
day in 70 F air, it doesn't feel warm or cold. But if
you jump into 70 F pool water you will feel cold, at least
until you "get used to" the water temperature (your body
might reduce blood flow to your extremities and skin to try
to reduce energy loss).
Air is a poor conductor. If you go out in 40 F
weather you will feel cold largely because there is a larger
temperature difference between you and your surroundings
(and temperature difference is one of the factors that
affect rate of energy transport by conduction).
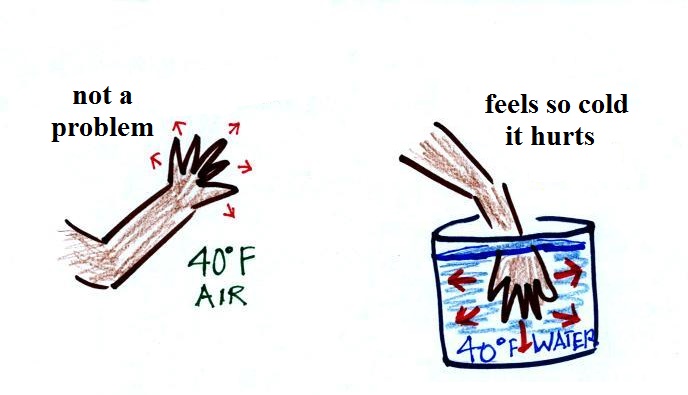
If you stick your
hand into a bucket of 40 F water, it will feel very cold
(your hand will actually soon begin to hurt). Keep
some warm water nearby to warm up your hand.
Water is a much better conductor than air. Energy
flows much more rapidly from your hand into the cold
water. I mentioned in class that I thought this might
be good for you. The reason is that successive
application of hot and then cold is sometimes used to treat
arthritis
joint pain (it used to work wonders on my Dad's knee).
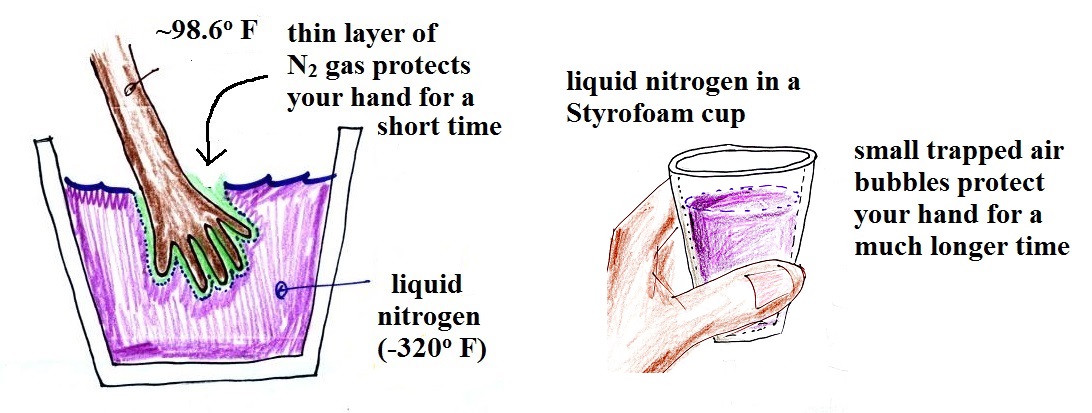
You can safely stick your hand into liquid nitrogen for a
fraction of a second. There is an enormous temperature
difference between your hand and the liquid nitrogen which
would ordinarily cause energy to leave your hand at a
dangerously high rate (which could cause your hand to freeze
solid). It doesn't feel particularly cold though and
doesn't feel wet. The reason is that some of the
liquid nitrogen evaporates and quickly surrounds your hand
with a layer of nitrogen gas. Just like air, nitrogen
is a poor conductor (air is mostly nitrogen). The
nitrogen gas insulates your hand from the cold for a very
short time (the gas is a poor conductor but a conductor
nonetheless). If you leave your hand in the liquid
nitrogen for even a few seconds it would freeze. That
would cause irreparable damage.
You can hold onto a Styrofoam cup of liquid nitrogen
longer. That is because the air can't freely move;
it's trapped in little air pockets in the foam. When
air is free to move, convection will begin to transport
energy at a more rapid rate than conduction alone.
A question came up in class a few semesters ago about
sticking you hand (or maybe just the tip of one finger) into
molten lead. I've never seen it done and certainly
haven't tried it myself. But I suspected that you
would first need to wet your hand. Then once you stick
it into the lead the water would vaporize and surround your
hand with a thin layer of gas, water vapor. The water
vapor is a poor conductor just like the nitrogen and oxygen
in air, and that protects your hand, for a short time, from
the intense heat. Here's a video
(and water does play a critical role)
Wind chill
Wind chill is a really good example of energy transport by
convection. As a matter of fact I'm hoping that
whenever you hear of energy transport by convection you'll
first think of wind chill. Wind chill is also a
reminder that our perception of cold is an indication of how
quickly our body is losing energy rather than an accurate
measurement of temperature.
Before we get into the details, here's a question:
It's 40
F outside,
the wind is blowing at 30
MPH,
and the wind chill
temperature is 28 F.
What temperature would you
measure with a thermometer?
Your body works hard to keep its
core temperature around 98.6 F. If
you go outside on a 40 F day (calm winds) you will
feel cool; your body is losing energy to the colder
surroundings (by conduction mainly). Your body
will be able to keep you warm for a little while
(perhaps indefinitely, I don't know). The 5
arrows represent the rate at which your body is losing
energy.
A thermometer behaves differently,
it is supposed to cool to the temperature of the
surroundings. Once it reaches 40 F and has the
same temperature as the air around it the energy loss
will stop. If your body cools to 40 F you will
die.
If you go outside on a 40 F day with 30 MPH winds your
body will lose energy at a more rapid rate (because
convection together with conduction are transporting energy
away from your body). Note the additional arrows drawn
on the figures above indicating the greater heat loss.
This higher rate of energy loss will make it feel colder
than a 40 F day with calm winds.
Actually, in terms of the rate at which your
body loses energy, the windy 40 F day would feel the
same as a 28 F day without any wind. Your body is
losing energy at the same rate in both cases (9 arrows
in both cases). The combination 40 F and 30
MPH winds results in a wind chill temperature of 28 F.
You would feel colder on a 40 F day with 30 MPH winds but
the actual temperature is still 40 F. The thermometer
will again cool to the temperature of its surroundings, it
will just cool more quickly on a windy day. Once the
thermometer reaches 40 F there won't be any additional
energy flow or further cooling. The thermometer would
measure 40 F on both the calm and the windy day.
Standing outside on a 40 F day is not an
immediate life threatening situation. Falling into
40 F water is, you might last 30 minutes (though you
might lose consciousness before that and die by
drowning).
Energy will be conducted away from your body
more quickly than your body can replace it. Your
core body temperature will drop and bring on hypothermia.
Be sure not to confuse hypothermia with hyperthermia
which can bring on heatstroke and is a serious outdoors
risk in S. Arizona in the summer.
Talk of how long you would last in 40 F water
reminds me of a page from the National Geographic
Magazine that lists some of the limits
of human survival. I'm trying to find a
complete citation.
We've covered a lot of material. It is
possible that there may still be some time left however. If
that turns out to be the case we'll get started on some of the Tue., Oct. 8 material on latent heat
energy transport.
Here's a summary of the main points
from today's class. Once you get more familiar with this
material you should be able to take out a couple of sheets of
blank paper, write down the 9 topic headings below (in bold font)
and fill in the details yourself.